Most of the geological processes we’ve discussed so far act to reshape our planet over thousands and millions of years, which means we rarely notice their effects on human time scales. However, some geological processes can be much more rapid, and these can pose great dangers to human life. Let’s briefly discuss some of these events, which you can think of as being examples of “instant geology.”
Discussion
“Instant Geology”
Before you read ahead, work in small groups or as a class to make a list of as many examples of “instant geology” — meaning geological processes that occur rapidly enough to pose dangers to us — as you can. Which of these processes are of particular concern in your local community? Have any of them affected your community in recent years?
This discussion is designed to help motivate students before we discuss various “instant geology” dangers in this section. Students are undoubtedly familiar with many geological dangers from news reports, and depending on where you live, some may pose particular risks in your local community.
Earthquakes, Landslides, and Tsunamis
Earthquakes, landslides, and tsunamis look very different (Figure 5.2.3–1), but they are closely related because earthquakes are one of the most common causes of landslides and tsunamis. These three related events are also all deadly, having caused some of the worst losses of life in human history. You are probably familiar with all three, but let’s make sure by defining them clearly:
- An earthquake is the sudden shaking of the ground caused by movement within Earth’s crust.
- A landslide occurs when rock, mud, or other debris comes sliding down a hill or slope .
- A tsunami is a huge, destructive wave that washes inland from the ocean (or, occasionally, on a large lake). Tsunamis are caused when the ocean is suddenly disturbed by an event such as an undersea earthquake, landslide, or volcanic eruption. This disturbance displaces (changes the position of) a large volume of water, causing a wave to move outward from that location.
Figure 5.2.3–1 – Earthquakes, landslides, and tsunamis are among the most destructive of all natural disasters.
Earthquakes
If you experience an earthquake, you’ll feel the ground shaking around you. Most earthquakes last for less than about 30 seconds (Video 5.2.3–2), but in a few cases the shaking can continue for up to a few minutes. If the earthquake is moderately strong, objects may begin to fall from shelves and windows may begin to break. If it is even stronger, entire buildings may collapse, and damage to electrical lines or gas lines may cause fires that may burn long after the earthquake itself is over. Given these events, it’s probably not too surprising that earthquakes have been responsible for some of the worst natural disasters in history.
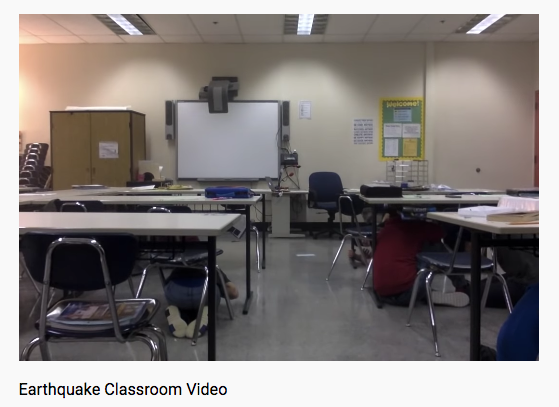
Earthquakes happen when rock in Earth’s crust suddenly breaks, causing the ground to lurch up, down, or sideways. Earthquakes usually occur along faults where rock on one side is being pushed in a different direction from rock on the other side. Typically, the rock will resist movement for many years, gradually increasing the stress on the rock. When the stress finally breaks the rock, the sudden movement causes the earthquake (Figure 5.2.3–3).
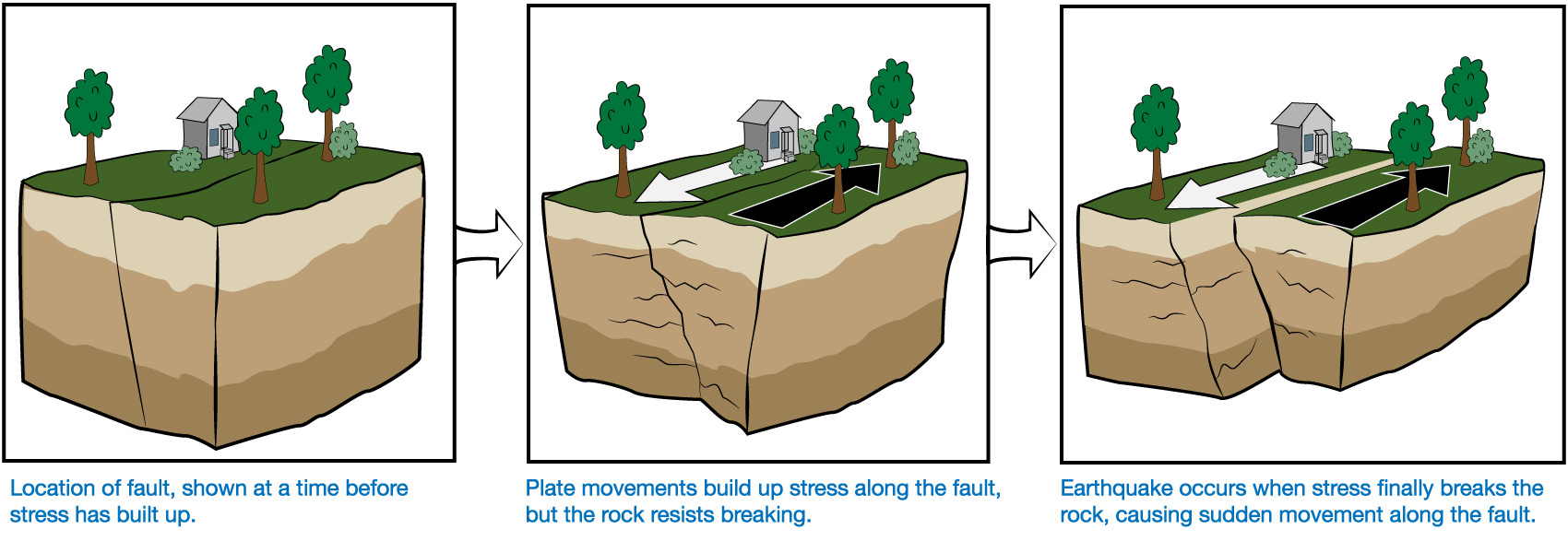
Faults are particularly common along plate boundaries, because those are places where two parts of Earth’s crust are moving relative to one another. One famous example is California’s San Andreas Fault (Figure 5.2.3–4), which marks a place where the plate carrying North America is moving southward relative to the plate carrying the Pacific Ocean and part of California.
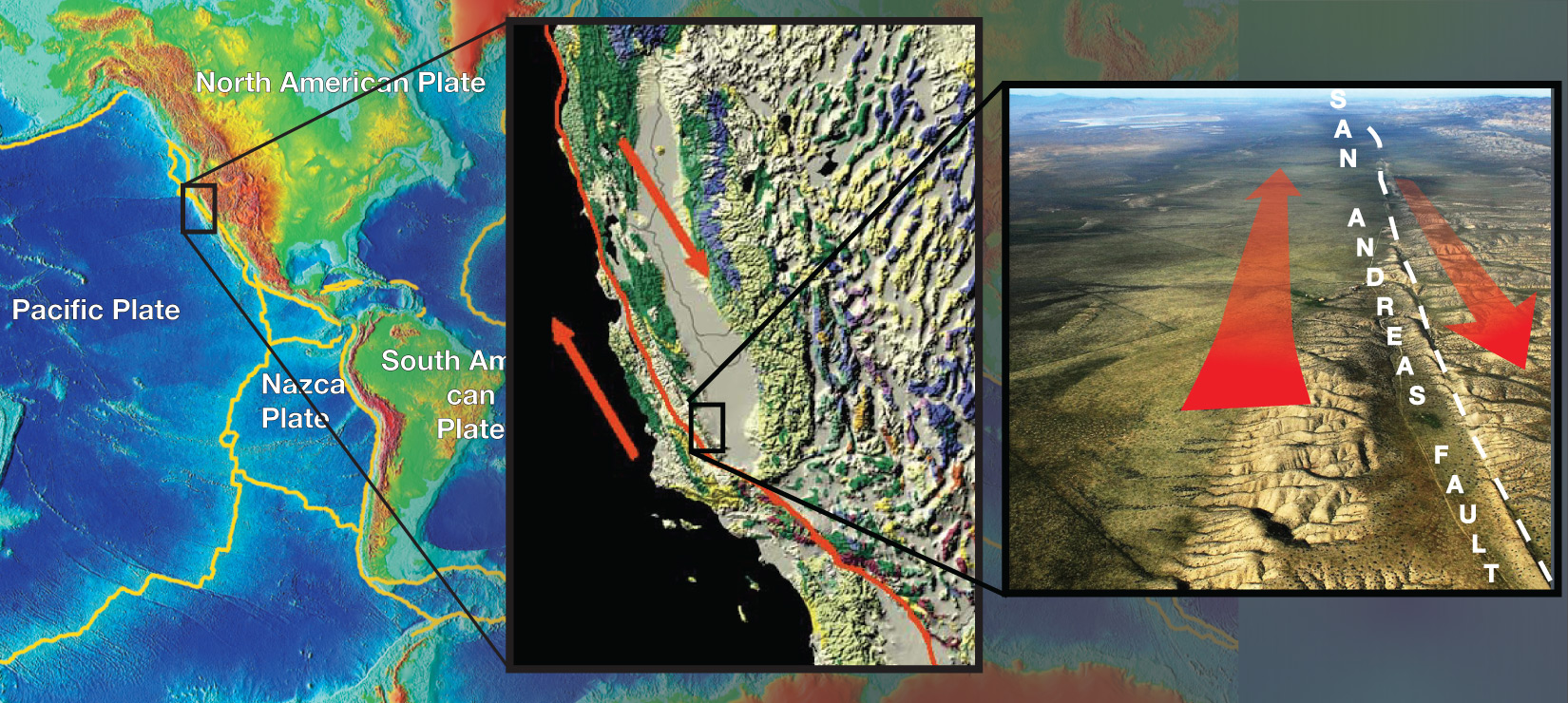
Although some earthquakes can be devastating, most are so weak that you wouldn’t notice them at all. We know they occur only because they are detected through the seismic waves they produce, which are detected with seismographs (see Figure 4.16). Scientists characterize the strength of an earthquake with a number called its “magnitude,” which is most commonly between about 1 and 10. The following table shows the general categorization of earthquakes by magnitude , including how often each category occurs:
Category | (Worldwide Average Since 1900) |
|
---|---|---|
Great | ||
Major | ||
Strong | ||
Moderate | ||
Light | ||
Minor | ||
Very minor | magnitude 1-2: 8,000 per day |
An earthquake’s magnitude is usually stated as a decimal to the nearest tenth. For example, the earthquake in Video 5.2.3–2 had a magnitude of 7.1.
What makes earthquakes so dangerous? Interestingly, earthquakes usually pose very little danger to people or animals outdoors. Unless you get hit by a rockfall or landslide caused by an earthquake, or a tsunami triggered by one, all that will happen is that you’ll feel the ground shake. The real danger from most earthquakes comes indoors, where a roof may collapse on you or you may be cut by shards of broken window glass. That is why the students in Video 5.2.3–2 got under the tables, which offered at least some protection in case the ceiling had collapsed.
Activity
What To Do in an Earthquake
What should you do if you ever experience an earthquake? Research earthquake safety recommendations ( here are the recommendations from the U.S. Geological Survey). Discuss them as a class, thinking both about what you would do if the earthquake hit while you were at school and what you would do at home. Then do the following.
- As a class, come up with a class safety plan, so that all students would know what to do if an earthquake occurred. Hold a drill to test out your plan, and refine it if needed after the drill.
- Each individual student should write up a short home safety plan, which you should then take home and discuss with your family, so that you and your family members will also be prepared if an earthquake strikes while you are at home.
This activity could be very valuable to students. If you live in an earthquake-prone area, you may already have an earthquake plan and conduct drills. But keep in mind that while some areas are more prone to earthquakes than others, an earthquake can strike almost anywhere. Therefore, it is good for all students to be prepared.
Large earthquakes are often followed by additional earthquakes called “aftershocks,” sometimes continuing for several weeks, which occur because rocks continue to break and move along the fault (or along nearby faults). Even though they are usually smaller than the initial earthquake, aftershocks can sometimes cause great damage. For example, in some cases the main earthquake may weaken a building’s structure but still leave the building standing, and an aftershock will then cause the building to collapse. This is of particular concern during rescue operations, because rescuers must always beware of aftershocks that could create further damage and danger.
Discussion
Recent Earthquakes
Discuss the following questions.
- Do research as needed to find out when an earthquake most recently occurred in your local region. What was the magnitude of the earthquake? Did it cause any damages, injuries, or deaths? Overall, how much of a threat would you say earthquakes pose in your region?
- Find news reports about significant earthquakes that have occurred around the world in the past couple of years. Where did the most damaging earthquakes occur? How many people were injured or killed? Discuss whether you think anything could have been done to have limited the deaths, injuries, and damages from these earthquakes.
This discussion should help students think about both local earthquake danger and about the impact of earthquakes around the world. For (2), students should recognize that while we can’t control the earthquakes themselves, the way in which we construct buildings and prepare for possible earthquakes CAN have a major impact on damages, injuries, and deaths.
Landslides
You’ve probably seen at least small landslides occurring, such as when rocks or mud slide down a hill after a storm. Large landslides can be much more devastating. Some have buried entire villages or towns, causing vast numbers of deaths (Figure 5.2.3–5).
Slideshow 5.2.3–5 – These slides show a few examples of landslides.
Most landslides are triggered by some event, such as an earthquake, volcanic eruption, or heavy rainfall . Rainfall is especially likely to trigger a “mudslide,” which is simply a landslide consisting largely of wet mud. Landslides can also be made more likely if trees and other vegetation have been stripped from a hillside, such as by a fire or by clear-cutting, because it removes roots and growth that would otherwise help prevent rock and soil from falling downward.
Activity
Local Landslide Danger
- Do research as needed to find out when a significant landslide (including mudslides or rockfalls) most recently occurred in your local region. Did it cause any damages, injuries, or deaths? Overall, how much of a threat would you say landslides pose in your region?
- Find news reports about significant landslides that have occurred around the world in the past couple of years. Where did the most damaging ones occur? How many people were injured or killed? Discuss whether you think anything could have been done to have prevented these landslides or make them less devastating.
- Now consider what you’ve learned from the first two questions and apply them to your local geography. Identify areas where a major rainstorm or an earthquake could potentially cause a landslide (or mudslide) in the future. Are there people living in harm’s way in those areas? If so, does it appear they’ve taken any steps to mitigate the danger? What further actions would you recommend for their safety?
This is very similar to the prior discussion of recent earthquakes, but focused on considering landslides and, in particular, any dangers they pose locally. Notes:
- (1) If you live in a very flat area where landslides are rare, ask students to interpret “local” as the nearest area in which landslides may occur.
- (2) Be sure that students consider the fact that, unlike earthquakes, landslides sometimes have human factors involved in their occurrence or severity. For example, deforestation or other denuding of hillsides or mountain slopes can make landslides more likely. Students should therefore consider whether human factors played any role in the landslide itself, along with considering what steps (locations of structures, etc.) might have prevented more damage and death.
- (3) This part of the activity can range from a simple one based on discussion of local geography to one in which students go out and actually find slopes prone to landslides, looking at buildings or other structures that might be affected if a landslide occurs.
Tsunamis
Take a look at Figure 5.2.3–6, which shows a huge rock (made out of coral) weighing more than 1,500 metric tons, sitting well inland on an island. The rock is clearly out of place, so how did it get there? The most likely answer is that it was lifted to this location by the type of giant wave known as a tsunami . Think of the power it must have taken to lift such an enormous weight from the sea to the island.
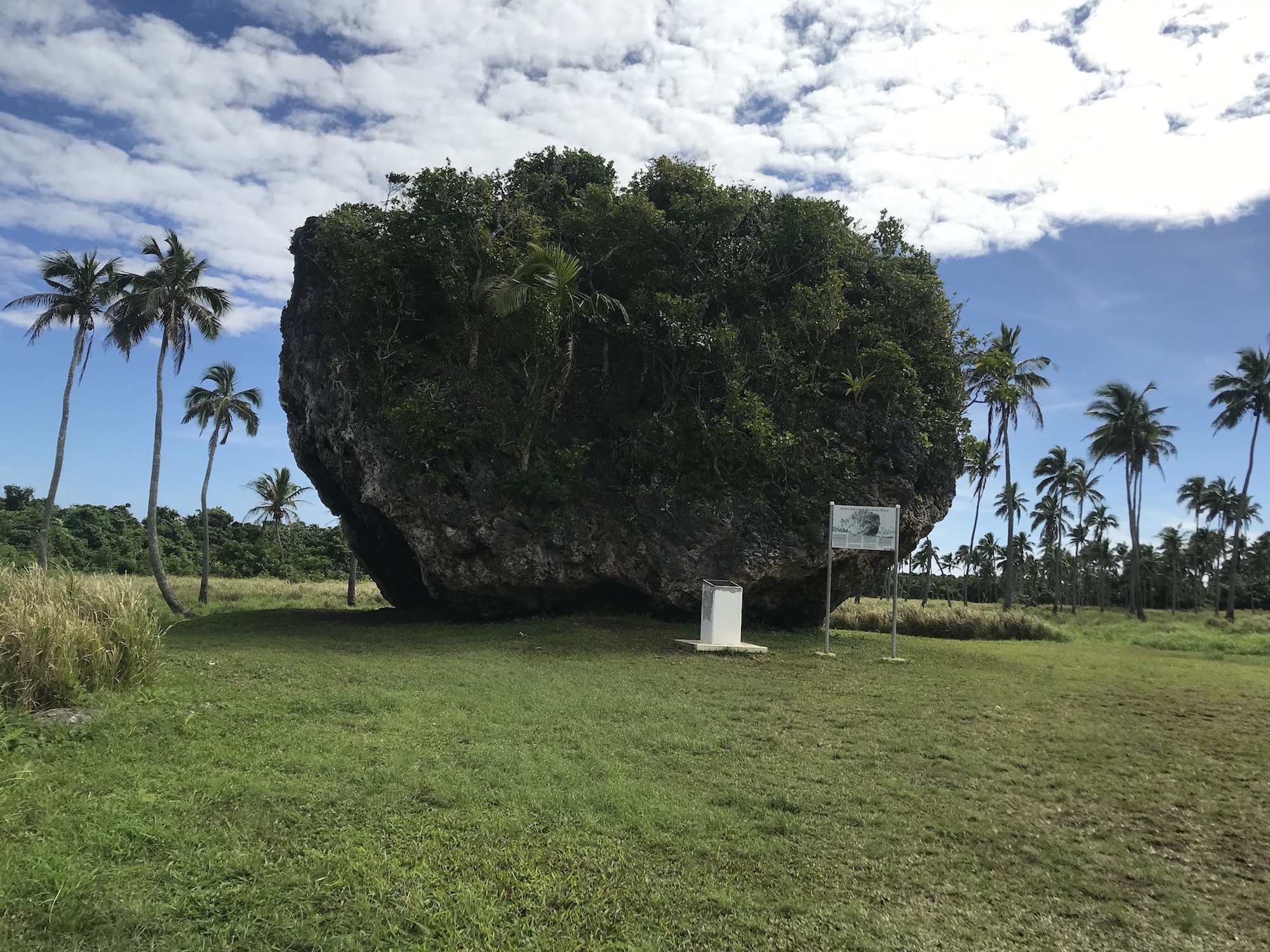
The fact that such a large rock could be moved by a tsunami should make their destructive power very clear. A tsunami is not just water rushing inland, but water packed with all the rock and debris it picks up along its path. In fact, many of the people killed in tsunamis are killed by being hit by this debris, rather than by drowning.
A further great danger with tsunamis is that while earthquakes and landslides generally cause damage only in a localized area, tsunamis can affect wide regions. In 2004, a huge tsunami killed more than 200,000 people, making it one of the one of the worst natural disasters in history. It was triggered by a huge earthquake (magnitude 9.1) off the coast of Indonesia, but the death and destruction did not stop there. Instead, the tsunami propagated across the Indian Ocean, killing people in 14 different countries, some thousands of kilometers away from where the earthquake struck (Video 5.2.3–7).
Video 5.2.3–7 - This video shows how the 2004 tsunami spread out and traveled across the Indian Ocean, after being triggered by an earthquake off the coast of Indonesia. Credit: NOAA.
Activity
Tsunami Warning System
One of the reasons for the large loss of life in the 2004 tsunami was that it struck most places without any warning. Yet, except very close to where the triggering earthquake occurred, it took from minutes to hours for the tsunami to reach the locations where it killed people. This means that, in principle, there should have been plenty of time to warn people. The problem was that, at the time, there was no warning system for tsunamis in the Indian Ocean. (In contrast, there was a warning system for Pacific tsunamis.)
- Working in small groups, briefly research how a tsunami warning system works.
- For the nearest coastline to your location, find out if a tsunami warning system is in place. If so, how long has it been in operation? If not, are there plans for installing one?
- Learn about a few of the most recent tsunamis around the world. Were lives saved by a tsunami warning system? Discuss why or why not.
This activity engages students in a very practical engineering problem, asking them to think about how a tsunami warning system can be built and how it can save lives. Be sure that students learn both about the buoys that detect ocean movement and how the buoy data is then used to determine if a tsunami is in progress and where warnings should be given.
Optional Video
“The Impossible”
If you have time and access to renting it, watch the movie “The Impossible,” which is based on the 2004 tsunami. The movie does a good job of showing and explaining why the event was so disastrous.
Warning: The movie can be quite disturbing, since it depicts real events in which huge numbers of people died. Use your personal judgment — and discuss with your family and your teachers — in deciding whether it is something you should watch.
VERY IMPORTANT: We call attention to this movie because we think it is educational and worth watching, but you should NOT assign it to all students, because of its disturbing nature as noted in the given warning. Most 8th graders are likely to be fine with it, but not all. Use your judgment in deciding whether and how strongly to recommend it for home viewing. If you decide to use it in class, be sure you alert parents and provide the option to opt-out of watching it.
- Note: As an alternative to renting a movie, there is a Youtube video with real footage that you can find here: https://youtu.be/llSqzpsuq7c. However, since this is real footage with real people dying, it may be even more disturbing than the dramatized version in “The Impossible” movie.
Volcanoes
When it comes to “instant geology,” it’s hard to top a volcanic eruption, which can suddenly transform an entire landscape. Figure 5.2.3–8 shows pictures of Mount St. Helens in Washington State (USA) before and after its 1980 eruption. Notice the dramatic change in the mountain’s shape.
Figure 5.2.3–8– Mount St. Helens, before and after its eruption on May 18, 1980. Credit: USGS.
Connections—Etymology
Volcano
The words volcano and volcanism have a very simple origin. In Roman mythology, Vulcan was the god of fire, and he was presumed to work in the active volcanos found in southern Italy. One of these volcanoes is even named directly for him: the volcanic island called Vulcano. Over time, the same name, slightly modified, came to be given to all volcanoes.
Volcanoes don’t always erupt with sudden outbursts. Some erupt almost continuously, with lava flowing out over a period of many years. This has been the case with Hawaii’s Kilauea volcano (Slide Show 5.2.3–9 and Video 5.2.3–10), and it is also the case with many undersea volcanoes. In fact, at any moment, there are usually at least a dozen or more volcanoes erupting somewhere around the world.
Slide Show 5.2.3–9 – Hawaii’s Kilauea volcano erupted almost continuously from 1983 to 2018. Scientists consider it likely that Kilauea will resume its eruption soon.
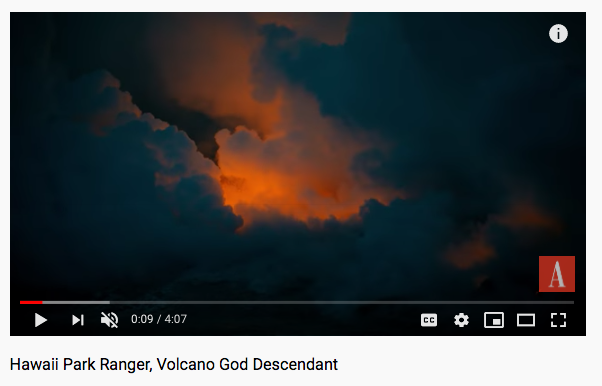
Activity
Virtual Volcano Trip
There are many places around the world where it is possible to see examples of recent or ongoing volcanic activity. Note that, in general, volcanic activity doesn’t necessarily mean an actual eruption is in progress. For example, most hot springs and geysers are the result of heating by underground molten rock, which means they also represent a type of volcanic activity.
For this activity, you will choose one site of volcanic activity and create your own “virtual field trip” report to present to your class either as a poster or as a slide show presentation. To do this:
- First, choose a site for your virtual trip. For example, you might choose to visit volcanic features of Hawaii, Iceland, Erta Ale (Ethiopia), Stromboli Island (Italy), Yellowstone National Park (USA), or many others. To help you choose, you can do a search on “volcanically active locations,” or you can visit this web site that gives a list of currently active eruptions.
- Once you’ve chosen your site, briefly research how you could in principle travel to the site, and what you would want to see once you arrived there. Assume you have 3 to 5 days available for your visit.
- Then create your presentation using images and text to describe what you see during your virtual field trip.
This main goal of this activity is for students to see that volcanism is a process that is always ongoing in at least a few places around the world. Students can work individually or in small groups. We recommend you ask each individual or group to choose a different location for their virtual field trip, so that in sharing the presentations, students will see a wide range of volcanic activity around the world.
Volcano Locations
We’ve already talked a fair amount about how volcanoes work. Recall that the molten lava that erupts from a volcano comes from a relatively small pocket of molten rock deep underground (see Figure 4.18). This means we expect to find volcanoes in places where underground melting is more likely, and you can probably guess that this means volcanoes are most common near plate boundaries. Indeed, we’ve already discussed why volcanoes are common where an ocean plate subducts under a continental plate, creating a deep ocean trench off the coast and a chain of volcanic mountains along the continent’s edge (see Figure 5.2.2–3a).
More generally, most volcanoes occur either in places where an ocean plate is subducting back into the mantle (under a continental plate or another ocean plate) or at mid-ocean ridges. However, there is one more major place where we find active volcanoes: over a mantle “hot spot” , which is a place where unusually hot mantle material is melting and rising upward to create volcanoes. Figure 5.2.3–11 summarizes the settings in which volcanoes commonly occur. Study the figure carefully, then use the discussion that follows to make sure you understand how this explains some of the ideas about geography that you learned in Chapter 4.
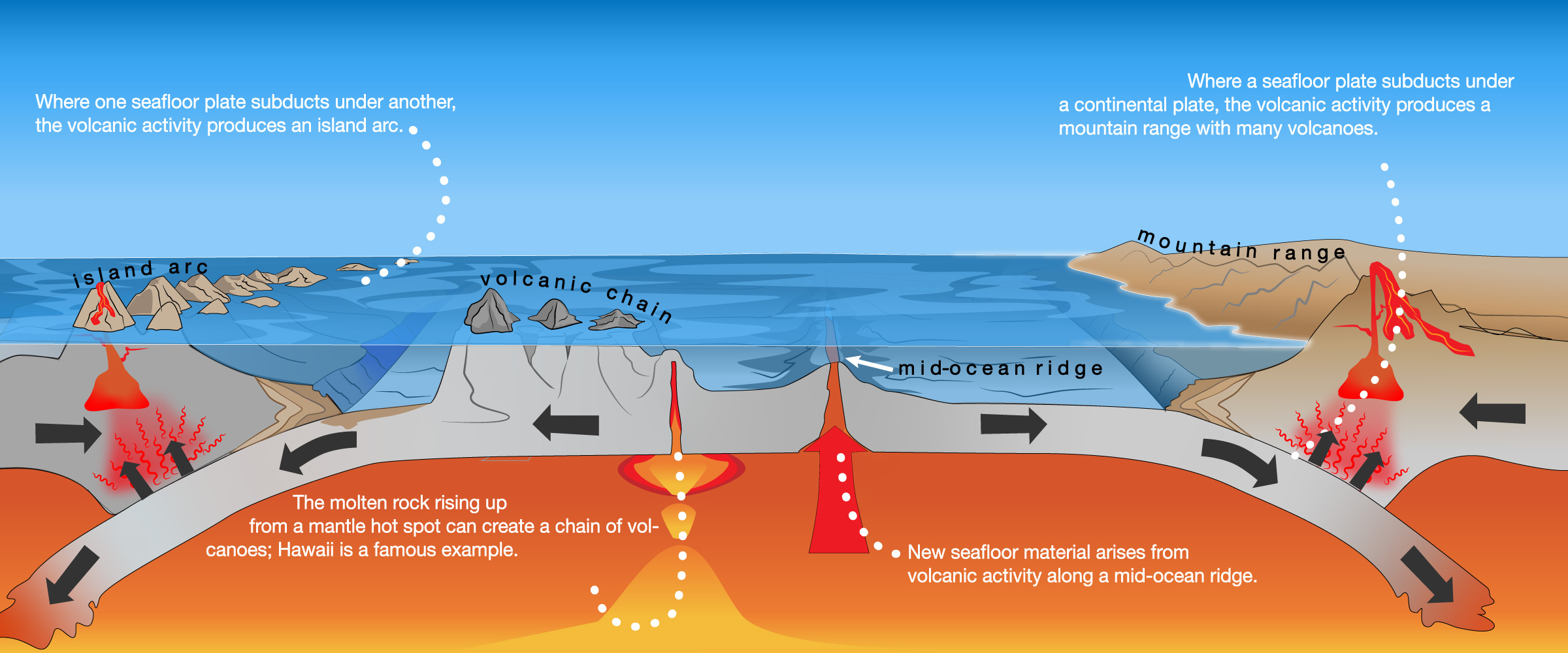
Discussion
Volcanism at Island Arcs and Mid-Ocean Ridges
Study Figure 5.2.3–11 carefully. Working in small groups, discuss the following questions that relate to the figure.
1. Look again at Figure 4.11. In an earlier discussion, you explained the association of the Peru-Chile trench with the Andes mountain range. Can you now also explain the association of trenches with island arcs and archipelagos? Give several examples.
2. Based on what you have already learned about seafloor spreading and what you see in Figure 5.2.3–11, what is happening to the plates on either side of a mid-ocean ridge? That is, are plates colliding, pulling apart, or sliding past each other?
This brief discussion will further help prepare students for understanding the full implications of plate tectonics in Section 5.3. Notes:
- (1): The association should be clear by comparing Figure 4.11 and Figure 5.2.3–11. Students should be able to cite numerous examples from the map in Figure 4.11 in which a trench is associated with an island arc (e.g., the Japan trench and the Japanese archipelago), and should then be able to explain how this relates to the volcanoes formed by the convergence of ocean plates in Figure 5.2.3–11.
- (2) This question should be fairly easy, as the figure shows clearly that plates are pulling part (divergent plate boundary) at the mid-ocean ridges.
Claim-Evidence-Reasoning Activity
The Hawaii Hot spot
Hawaii is actually part of an even longer chain of islands and seamounts (which are essentially “islands” that are entirely below the sea surface) shown in Figure 5.2.3–12. Let’s look at some of the evidence that leads to the idea that this chain was formed by a mantle hot spot.
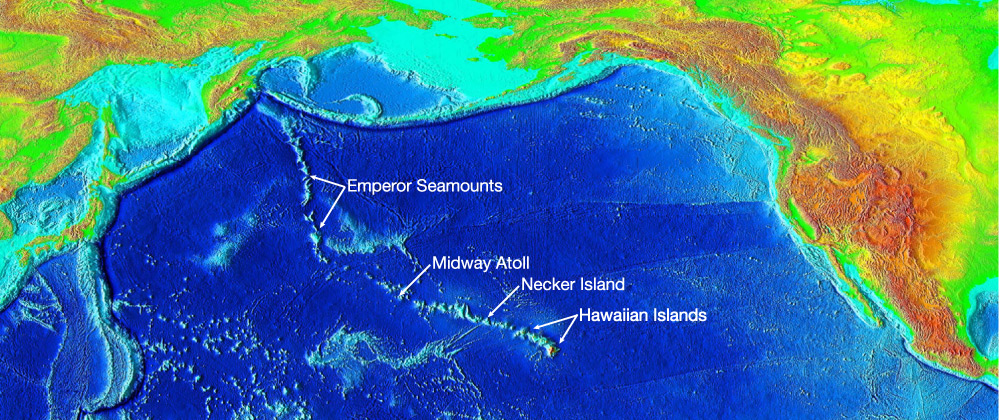
1. Compare what you see in Figure 5.2.3–12 against the map of plate boundaries shown in Figure 4.19. Is Hawaii on or near a plate boundary?
2. Use evidence and reasoning to support the following claim:
Claim: The volcanic islands of Hawaii must have been formed by molten rock rising up
from a mantle hot spot.
3. The big island of Hawaii has the tallest volcanic mountains of any of the islands and also shows relatively little evidence of long-term erosion. But as you go northwest along the chain, the island mountains become lower (eventually becoming seamounts) and show more and more evidence of having been eroded away. This suggests that the islands are increasingly older as you go northwest along the chain. How could you use absolute dating techniques to test this hypothesis?
4. The following table gives data on the approximate age and distance from the big island of Hawaii for several islands and seamounts in the chain. Using graph paper or computer software, plot these data on a graph in which you put distance on the x-axis and age on the y-axis. In words, summarize the trend you find.
*The given age is the approximate average for each island, except for Hawaii which is given age 0 to indicate that parts of it are still forming. |
---|
5. Based on what you’ve learned, use evidence and reasoning to support the following claim:
Claim: The Hawaiian islands formed one after the next as the plate that carries them has
moved over the mantle hot spot.
6. Bonus: The hot spot is still active, since that is what accounts for the activity of the Kilauea volcano on the southeast portion of the big island of Hawaii. Assuming that the hot spot is large enough to support more than one eruption at once, and that other eruptions might form new islands, answer the following questions:
a. In which direction from Hawaii would you expect the next island in the chain to eventually emerge?
b. Look up the location of the “Loihi seamount.” Is it where you would expect for the next island in the chain?
c. If Loihi keeps growing at its current rate, when will it become a new island in the chain?
d. If you were offered a chance to make an investment in future beachfront real estate on Loihi, would you take it? Why or why not?
7. Bonus: Recall from Section 4.3 that, because Mars is smaller than Earth, we expect Mars to have had less total volcanic activity than Earth. Yet Mars has several volcanoes larger than any on Earth, including Olympus Mons, the largest mountain in the solar system. Suggest a hypothesis by which Martian volcanoes grew so large by assuming that Mars lacks plates that can move around.
This CER activity investigates Hawaii as the classic example of hot spot volcanism. Notes:
- (1) Hawaii and the associated chain is not near a plate boundary.
- (2) To support this claim, students should note that the islands are clearly volcanic in origin (e.g., they have already learned about the active Kilauea volcano), and this tells us that there is molten rock rising up from the mantle to the surface. Since a hot spot is defined as a place within a plate where molten rock is rising up, Hawaii must be atop one.
- (3) If the hypothesis is correct, then each island along the chain should be increasingly old in absolute age, which you should be able to (and can) test through radiometric dating of the lava rock.
- (4) The graph should look something like the graph shown below. Encourage students to label their graphs clearly. In words, the trend is that increasing distance from Hawaii goes with increasing age.
- (5) The data showing that age increases with distance from Hawaii makes clear that the islands are forming in sequence, and the nearly straight-line pattern implies that the plate must be moving relative to the hot spot.
Note: We expect students to recognize only the relative motion of the plate compared to the hot spot, which is separate from the question of whether it is the plate or the hot spot that is moving. In fact, other evidence indicates that it has been the plate moving throughout the formation of the Hawaiian chain, explaining its nearly straight line. The kink in the path that occurs at the beginning of the Emperor Seamount chain is not fully understood, but some evidence suggests that prior to when the kink arose (about 40 million years ago), the hot spot had also moving; hence, when the hot spot motion stopped, the direction of the chain changed. - (6) The path of the islands suggests the next island would form to the southeast of Hawaii, and that is indeed where the Loihi seamount is located. At its current growth rate, Loihi could become an island in about 100,000 years. Part (d) this question addresses investing in future beach real estate, for which students should think about the 100,000 year time frame and realize that this would not be a smart use of money today.
- (7) This is a challenging question, because no one knows the full reason for the huge size of the Martian volcanoes. However, because Mars shows no evidence of having ever had plate tectonics, a reasonable hypothesis is the following: Since Mars lacks plates or plate boundaries, the molten rock that powered Martian volcanoes must come from mantle hot spots. On Earth, such hot spots can live for tens of millions of years, but no single volcano becomes “oversize” because plate motion makes a chain like that of Hawaii. Now, imagine what Hawaii would be like if the plate was stationary over the mantle hot spot: In that case, instead of a chain of islands, a single island would have continued to grow the entire time. That may be what happened on Mars. In other words, hot spots may be similar on Earth and Mars, but the lack of plate motion on Mars allowed individual volcanoes to grow much larger.
Connections—Culture
Pele
Study of Hawaiian legends indicates that ancient Hawaiians recognized the geological evidence indicating that the island chain forms an age sequence. In the traditional Hawaiian religion, Pele is the goddess of volcanoes and fire who created the Hawaiian islands. Legend holds that Pele was being chased by her sister Namaka, and her journey of escape led her to one-by-one create the volcanoes that made the islands, explaining the sequence of ages.
You might wonder: Given that they did not have modern dating techniques, how did the ancient Hawaiians know that the islands formed an age sequence? No one knows for sure, but the most likely answer is that they inferred it from careful observations of the islands. For example, they surely recognized the similarities between the active volcanic craters on the island of Hawaii and the inactive craters on other islands, which would have enabled them to figure out that those craters represented extinct volcanoes. They probably also recognized that erosion could grind away at mountains, so the fact that the islands form a sequence showing more and more erosion as you journey northwest would likely also have led them to conclude they formed an age sequence.
Volcano Danger
Volcanic eruptions can be deadly to people and wildlife living nearby. The most obvious danger comes from the flowing hot lava of an eruption, which will destroy anything in its path. But other dangers can be even worse.
Clouds of hot gas and debris from the volcano can rush downhill over a much larger area than a lava flow, sometimes at speeds of a few hundred kilometers per hour — making them impossible to outrun, even in a car. These “killer clouds” are often responsible for most of the deaths after a volcanic eruption. For example, they were the primary killers in the famous eruption that destroyed the cities of Pompeii and Herculaneum in 79 A.D. (Figure 5.2.3–13).
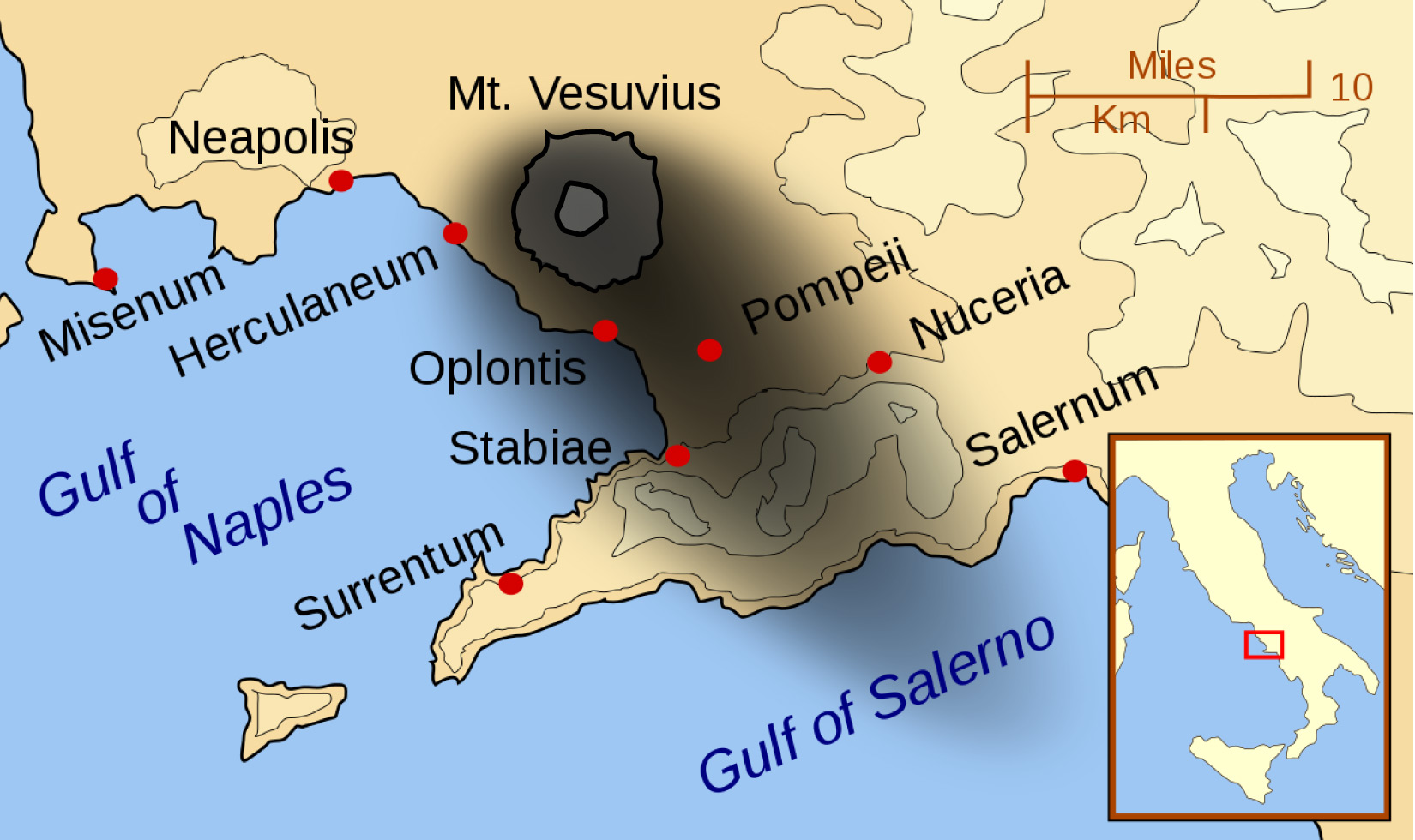
Other hazards to people living near a volcano include toxic gases and landslides or mudslides triggered by volcanic activity. These hazards can sometimes occur even when there is not a full eruption, since active volcanoes often release gas or shake with earthquakes.
Volcanic eruptions can occasionally affect people much farther away from them. For example, an eruption in Japan in 1792 triggered a landslide that sent much of Mt. Mayuyama tumbling into the sea, where it generated a tsunami that killed an estimated 15,000 people living more than 20 kilometers away (Figure 5.2.3–14).
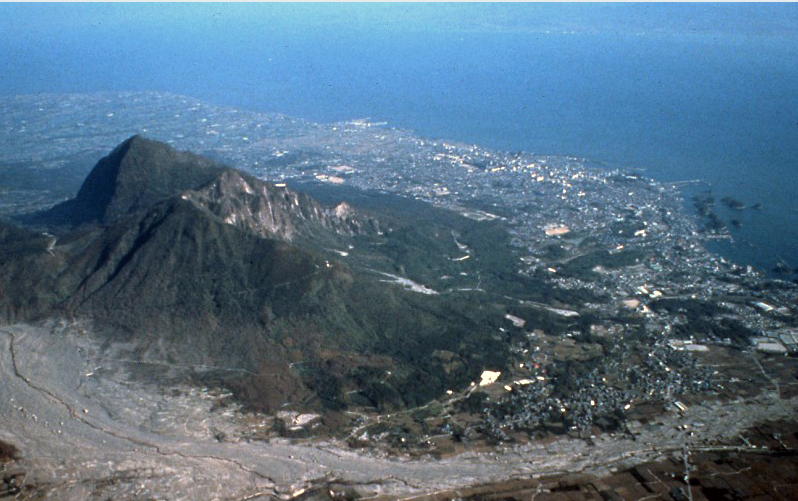
On rare occasions, volcanic eruptions can be so large that they affect the global climate. One famous case was the eruption of Mt. Tambora (Indonesia) in 1815. This eruption released an enormous quantity of small particles into the upper atmosphere, where they drifted around the world. Because these particles reflect sunlight, they caused the entire world to cool, explaining how the following summer (1816) came to be known in Europe as the “year without a summer.” More recently, the 1991 eruption of Mt. Pinatubo (Philippines) caused the largest release of small particles into the upper atmosphere of the 20th century (Figure 5.2.3–15). Scientists measured global cooling of about 0.5°C for more than a year following this eruption.
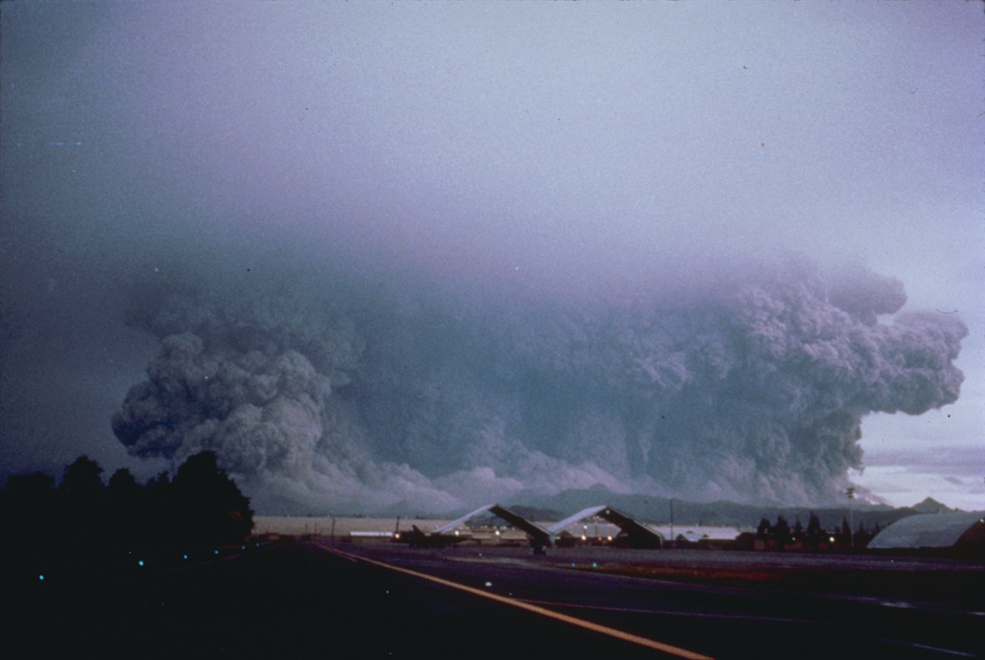
Activity
Historic Eruption
Many volcanic eruptions have had significant impacts on humans during recorded history, including large loss of life. Here are just a few famous examples: the eruption of Mt. Vesuvius in 79 A.D., which destroyed the cities of Pompeii and Herculaneum; the 1815 eruption of Mount Tambora (Indonesia); the 1883 eruption of Krakatoa (Indonesia); the 1902 eruption of Mount Pelée (Martinique); the 1980 eruption of Mount St. Helens; the 1985 eruption of Nevado del Ruiz (Colombia); the 1991 eruption of Mount Pinatubo (Philippines); and the 2010 eruption of Eyjafjallajokull (Iceland). Choose either one of these eruptions or some other famous eruption to research. Then create a poster or slide show presentation about the eruption and its consequences to humans.
This activity is designed to help students become more familiar with some of the famous volcanic events in history. As usual for these presentations, students can work either individually or in small groups, and we encourage you to make sure that each student/group chooses a different eruption to investigate, so the class as a whole will benefit from the more diverse set of presentations.
Ancient Eruptions
How large can a volcanic eruption be? Geologists have found evidence that Earth has on occasion experienced eruptions far larger than any that have occurred during recorded history.
One example is at Yellowstone National Park (USA), which sits atop a huge mantle hot spot, explaining the many hot springs, geysers, and other volcanic features you can see in the park (Slide Show 5.2.3–16). Much of the national park is actually a giant volcanic crater (sometimes called a “caldera”) that had its last major eruption about 620,000 years ago. That eruption was estimated to be about 1,000 times as large as the 1980 eruption of Mount St. Helens (see Figure 5.2.3–8). Such an eruption today would be devastating to a large region of the United States and might also significantly affect the global climate. Fortunately, there is no evidence that Yellowstone will suffer another major eruption anytime soon, and long-term averages suggest the next major eruption is still at least 100,000 years away.
Slide Show 5.2.3–16 – Take a quick tour of some of the volcanic features you could see on a visit to Yellowstone National Park.
Even larger eruptions seem to have occasionally occurred in the more distant past. The primary evidence comes from what geologists call “large igneous provinces,” which simply means large areas covered by igneous rock that came from volcanic eruptions. Absolute dating techniques (primarily radiometric dating) allow geologists to figure out when the eruptions that created these regions occurred. One of the largest of these “igneous provinces” is known as the “Siberian Traps” (Figure 5.2.3–17), which formed as the result of a series of eruptions that began a little more than 250 million years ago and lasted for more than 1 million years.
Figure 5.2.3–17 – The Siberian Traps are a “large igneous province,” meaning a large region covered by igneous rock from volcanic eruptions. The eruptions that made the Siberian Traps occurred about 250 million years ago.
Eruptions as large as those that formed the Siberian traps can have severe global consequences. To see how severe, consider the following questions.
Discuss the following questions with a classmate. Then click to open the answers to see if they agree with what you came up with.
1. Look back at the geological time scale in Figure 4.24. What major event occurred at about the same time as the eruptions that formed the Siberian traps?
The Siberian traps formed about 250 million years ago, and Figure 4.24 shows that this time also marks the “greatest known mass extinction” . More specifically, careful study of the fossil record shows that close to 90% (perhaps even more) of all the species living on Earth before the eruptions were extinct after them. In other words, something killed off most of the life on Earth at that time, and the eruptions are the most obvious suspect as the trigger for this huge mass extinction event.
2. Again using Figure 4.24, explain why the major event of 250 million years ago is often called the “end-Permian” extinction.
Figure 4.24 shows that this extinction event marks the division point between what geologists call the Permian and the Triassic periods. Therefore, the extinction occurred at the end of the Permian period, making it the “end-Permian” event (sometimes instead called the “Permian-Triassic” event). Note: Recall that different time periods are named based on changes that geologists observe in the fossil record. The sudden extinction of most of Earth’s species creates a major change in the fossil record, which is why this time marks the end of one period and beginning of another.
Notice that, in the answer to question 1 above, we said the eruptions were the likely “trigger” for the end-Permian mass extinction. We say this because the eruptions themselves could not have caused such devastation. After all, the lava flows that made the Siberian traps occurred only in Siberia, so they could not have killed life all around the world. Instead, scientists assume that the eruptions started a chain of other events that killed off most of the life. There is still considerable scientific debate about the details, but the leading hypothesis holds that the volcanic activity ultimately led to enormous global warming — far more than we humans are causing today. According to this hypothesis, most species were unable to adapt to the rapidly changing climate and were therefore driven to extinction.
Optional Activity
Research the End-Permian Extinction
Do some research to learn more about the hypothesized causes of the end-Permian mass extinction. Prepare a short written or oral report in which you detail at least one hypothesis for how this extinction event occurred. Be sure to discuss both the strengths and weaknesses of the hypothesis.
Impacts
Recall that Earth has been hit numerous times throughout its history by asteroids or comets large enough to make impact craters. Figure 5.2.3–18 shows Meteor Crater (Arizona, USA), which formed from an asteroid impact that occurred about 50,000 years ago. One look at this crater should convince you that it represents another form of “instant geology,” and that the impact must have been devastating to any living creatures that were in its vicinity.
Larger impacts have occurred in the more distant past, some with extreme consequences. For example, as we’ll discuss in Section 5.4.2, one very large impact is thought to have been the cause of the mass extinction that wiped out the dinosaurs. However, in terms of “shaping Earth’s surface” — which is our topic in this section — impacts have played a relatively minor role, because impacts large enough to make impact craters occur quite rarely compared to the geological processes that are intrinsic to Earth itself.
Activity
Local Geological Hazards
No matter where you live on Earth, there are at least some potential geological hazards to your community, some of which you may have already identified in an earlier discussion. Start by making a list of significant local hazards. Then break into small groups, with each group addressing one hazard, and do the following:
- Learn all you can about the danger. For example, what is the cause of the danger, and how does its geology work?
- Learn what is currently being done to help protect your community from the geological danger.
- Within your group, discuss whether you think the danger is being addressed adequately, or whether more should be done to protect the community. If you think more should be done, come up with specific ideas about what that should be.
- Put together a presentation for the rest of your class in which you summarize what you’ve learned and explain any actions you recommend to protect your community.
This activity is fairly open-ended, so you can spend anywhere from very little time to multiple class periods on it. It should feel very relevant to students, because of the potential impacts on them, their families, and their community. If you live in a place where there are larger geological dangers — such as a region near a volcano, or prone to earthquakes, or a coast with significant tsunami danger — you might wish to have students develop detailed proposals for enhancing community safety. With regard to the presentations in step 4: If you want to use minimal class time, then you can ask students to put together written or poster presentations. If you want to engage in more discussion, ask each group to make a 5- to 10-minute oral presentation to the class.