We have already talked a fair amount about “energy,” noting that there are two main sources of energy that drive the processes we seek to understand in Earth system science. But what exactly is energy? In the coming chapters, we’ll need to have a more precise way of talking about energy in order to look deeper into Earth’s systems. We’ll also need to understand the distinction made in science between energy and “power.”
Brief Definitions of Energy and Power
You’ve probably learned about energy in your past science courses, in which case you will know that it’s surprisingly difficult to come up with a simple definition of “energy.” In fact, if you look it up, you’ll find that different dictionaries (or other sources) define energy in a variety of different ways. Nevertheless, for most purposes, we can define energy in science in this fairly broad way:
- Energy is something that can make matter move or heat up.
Discussion
The Definition of Energy
1. Working in small groups or as a class, consider the following sentences that use the word “energy” in different contexts. In each case, explain how the usage of the word “energy” meets the definition given above.
- Evaporation is driven by the energy of sunlight.
- Earth’s interior contains a lot of energy.
- It takes energy to run a refrigerator.
- I’m a high-energy person.
- I’ll have to put a lot of energy into thinking about this problem.
2. Think of at least three more sentences using the word “energy” that you might hear in everyday life. Again, decide whether the usage in each case meets the definition above.
For the first question, students will hopefully realize that the definition works for these sentences as follows:
- Evaporation is driven by the energy of sunlight. This meets the above definition, because heat from sunlight causes water molecules to move more rapidly until they can escape (evaporate) from the liquid phase into the gas phase.
- Earth’s interior contains a lot of energy. This meets the definition because even when nothing is moving, the internal heat of the Earth could in principle cause something to move, as it does when it drives volcanoes or earthquakes (for example).
- It takes energy to run a refrigerator. Refrigerators run by circulating a refrigerant, which involves motion. They also release heat in the process of keeping the refrigerant cool, so they also involve making matter heat up.
- I’m a high-energy person. This arguably also meets the definition, because it generally means a person who moves a lot.
- I’ll have to put a lot of energy into thinking about this problem. The connection between thinking and motion might be less obvious, but on a biological level, thinking involves the firing of neurons in the brain, which does indeed involve motion.
For #2, answers will vary depending on the sentences that students come up with, but if they look deep enough, they can probably see a connection to the above definition of “energy” in all cases.
In everyday life, we often use the terms energy and power interchangeably, but in science the term “power” has a slightly different meaning:
- Power is the rate at which energy is being used, released, or generated.
As a simple example of the difference between energy and power, think about a light bulb (Figure 4.41). A particular light bulb will be labeled with the amount of power that it uses when turned on (for example, 15 watts). This amount of power is always the same for that particular light bulb. In contrast, the amount of energy the light bulb uses depends on how long you leave it on. If you leave it on for 10 minutes, it will use twice as much energy as if you leave it on for 5 minutes.
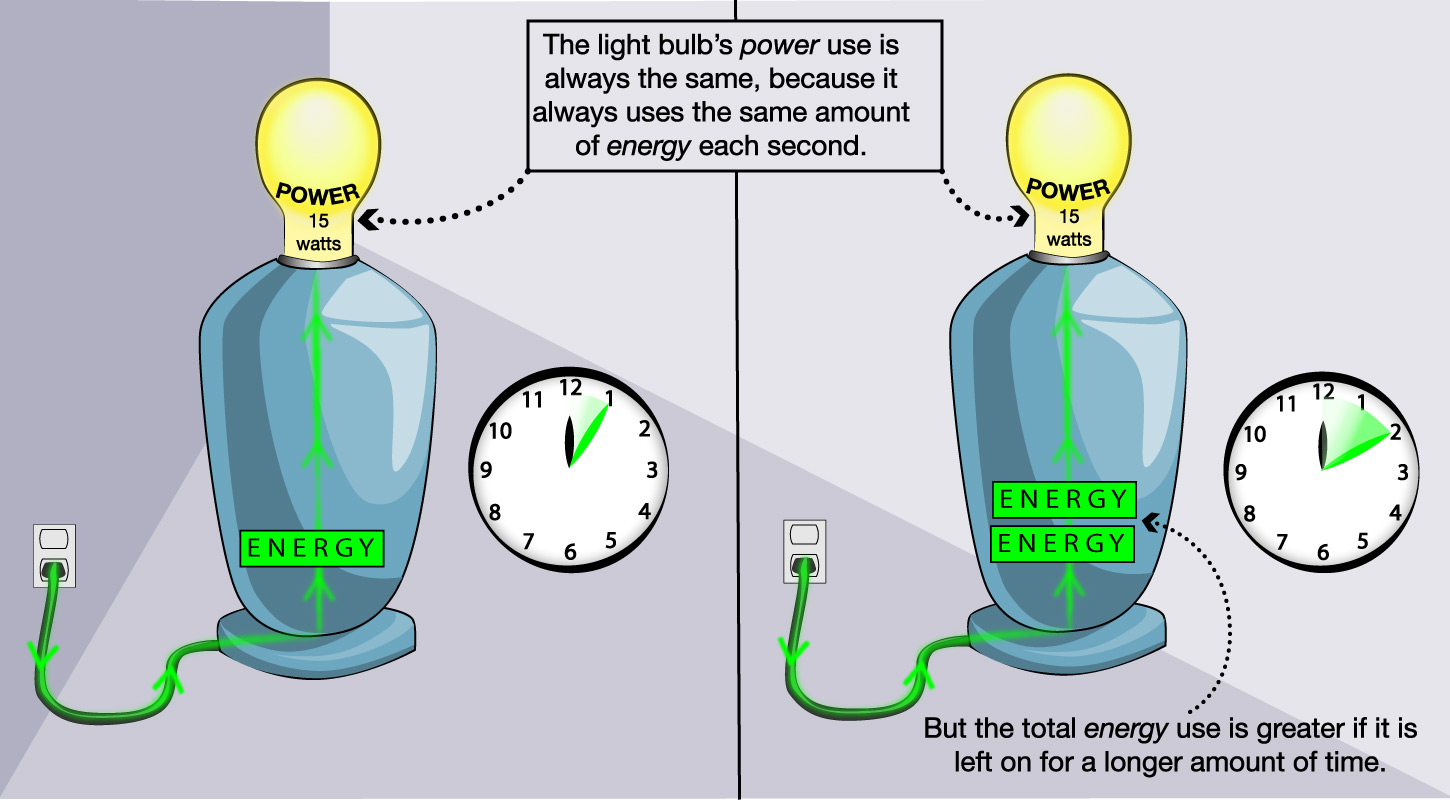
Discussion
The Distinction Between Energy and Power
Discuss the following questions to make sure you understand the distinction between energy and power.
- When you eat an apple, does it provide your body with energy or power?
- Suppose you sprint as fast as you can for a 100-meter dash, then later go out jogging at an easy pace for 5 kilometers. Which event requires more power? Which requires more energy? Explain.
- When your family receives a bill for your electricity use over the past month, is the bill for energy or for power?
- Consider an electrical surge (such as the kind that can be caused by lightning) that damages an appliance. Did the damage occur because of too much energy or too much power?
This discussion should clarify the difference between energy and power. Notes on the answers.
- Like any food, the apple provides a certain amount of energy that your body can then use for metabolism or moving muscles or similar. Note: In an “I was wondering” below, we discuss the fact that the available energy content of food is generally measured in either calories (in the U.S.) or kilojoules (in the rest of the world). Students are probably familiar with these labels, but likely did not previously know how they related to energy.
- The sprint requires more power, because you are using energy at a faster rate during the sprint. The jog requires more total energy, because even though the jog involves less power, the fact that you are jogging for a much longer time more than makes up for that in terms of the total energy expended.
- You are billed for the total amount of energy that you used for your electrical appliances over the past month.
- The best answer is power, since the surge represents a very high rate of energy going through the system, and power is the term we use for the rate of energy flow. Note, however, that “too much energy in a short time” would therefore also be a valid answer to this question.
Note: The last question illustrates a sematic subtlety that may be worth discussing with your students:
- When talking about total energy, we can validly use only the term “energy” (not “power”).
- But when talking about power, we can often interchange the terms power and energy, as long as the rate context is clear.
For example, a 25-watt light bulb requires more power than a 15-watt light bulb, but this also means it requires more energy for any given amount of time.
Basic Types of Energy
In everyday life, we talk about many different kinds of energy. For example, we talk about the energy we get from food, the energy needed to drive a car, the energy of sunlight, electrical energy, the internal energy of the Earth, and more. Looking more deeply, however, scientists have found that all forms of energy can be classified into just three basic types:
- Energy of motion, which is more formally called kinetic energy (kinetic comes from a Greek word meaning “motion”).
- Energy carried by light, or radiative energy (the word radiation is often used as a synonym for light).
- Stored energy, which is more formally called potential energy , a name it gets because it has the potential to later be converted into energy of motion or radiative energy.
Energy can change its form among these three types (Figure 4.42), but it can never be created or destroyed (an idea embodied in what is called the law of conservation of energy ).
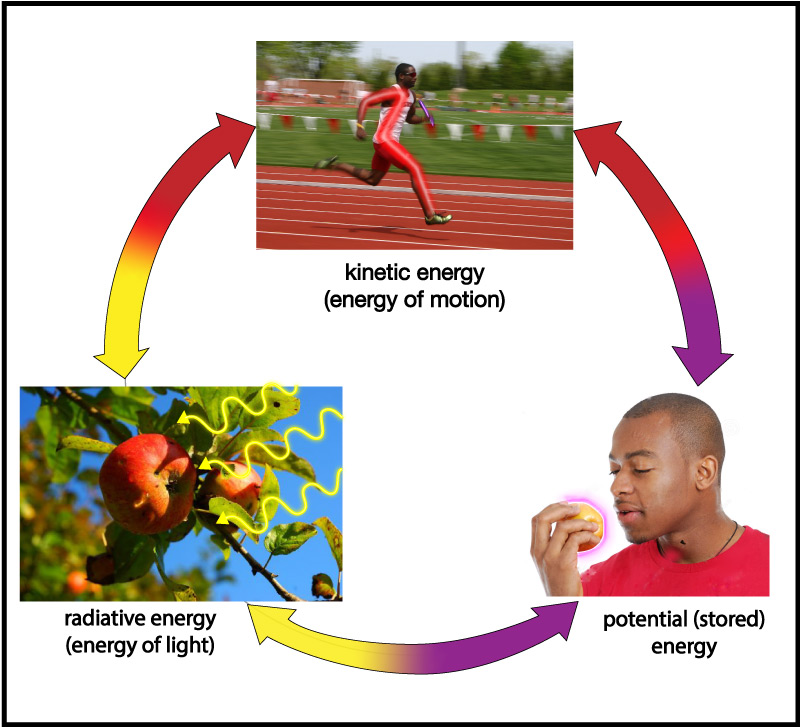
Discussion
Light, Sight, and Motion
You’ve learned that energy is something that can make matter move (or heat up). You also know that vision (sight) comes from light entering our eyes. In what way does the light that enters our eyes cause matter to move?
This optional discussion, which can be done in small groups or as a class, is designed to show that the definitions we’ve discussed are indeed universally valid. In this case: Vision occurs because light that enters our eyes is actually absorbed by cells in our retinas (rods and cones) that then transmit signals to the brain. This process involves physical movement of molecules within the cells. In other words, the radiative energy of light is converted into kinetic energy of molecules within cells of our eyes, and from there converted into the signals that go to the brain.
Energy Drivers of the Earth
In high school and beyond, you will learn much more about these basic types of energy. Here, let’s just look at how the two main energy drivers for Earth fit into these basic types:
- The first major driver, energy from the Sun, is radiative energy, since that energy reaches us in the form of sunlight.
- The second major driver, Earth’s own internal heat, is less obvious. However, as you may recall from physical science, on a molecular level heat is created by the motions of atoms and molecules: the higher the temperature, the faster the atoms and molecules in any substance — such as air or water or a rock — are moving (Figure 4.43). In other words, Earth’s internal heat is actually a form of kinetic energy, sometimes called thermal energy .
Figure 4.43 – Temperature is a measure of the average kinetic energy of atoms or molecules.
Temperature and Heat
There’s an important subtlety here, which is that temperature and heat, or thermal energy, are not quite the same thing. The following discussion should help you understand this idea.
Discussion
Oven and Water
Work in small groups or as a class to answer the following questions about an oven turned on as high as it goes and a pot of boiling water.
- Which has a higher temperature: the hot oven or the boiling water?
- Suppose you open up the hot oven, so that some of the hot air spills out at you. Will it burn you?
- What would happen if even a tiny bit of boiling water spilled on you? (Don’t try this!!!)
- Based on your answers so far, which would you say transfers more heat (thermal energy) to your skin if it touches you: the boiling water or the oven air?
- Try to fill in the blank: The total heat (thermal energy) content of the boiling water is much greater than that of the oven air because water has a much higher ________ than air, even though the oven air has a higher temperature than the boiling water.
This discussion should clarify the difference between temperature and heat (thermal energy). Notes on the answers.
- Students should either recall or look up the fact that the boiling temperature of water is 100°C (212°F). From their own experience with ovens at home, they should know that a typical home oven will have a maximum setting that is much higher than this.
- Students have almost certainly opened hot ovens many times without being burned. In fact, they’ll probably also realize that as long as they don’t touch anything inside the oven, it is even safe to reach in momentarily.
- Hopefully students are aware that even a small amount of boiling water can cause a severe burn; if they don’t know this, be sure you explain it, since it is an important safety lesson.
- The fact that a small amount of boiling water can cause a severe burn while you can safely be exposed (for a short time) to the oven air tells us that the boiling water must be transferring much more heat (thermal energy) to the places where it touches your skin. Note: On a molecular level, the transfer of heat occurs as molecules from the air or water bang into your skin, transferring their energy to your skin.
- Students may need to discuss this one for a bit, but hopefully will converge on recognizing that the answer is density. The boiling water can transfer much more thermal energy to your skin because it is so much more dense than the oven air, even though the oven air has higher temperature. Note that the molecular level of this is discussed and illustrated in Figure 4.44 below.
Figure 4.44 illustrates what you should have found with the above discussion. The higher temperature of the oven air means that these molecules are moving faster and therefore strike your skin harder, on average, than the molecules in the boiling water. However, the much higher density of water means that many more molecules are hitting your skin (in the places where the water touches you). While each individual molecule hitting your skin from the boiling water transfers a little less energy than a molecule of air from the oven, the sheer number of water molecules hitting you means that more thermal energy is transferred to your skin. To summarize:
- Temperature is a measure of the average kinetic energy of the atoms and molecules in a substance.
- The thermal energy content (or heat content) of a substance is the total amount of kinetic energy of all its particles in a substance.
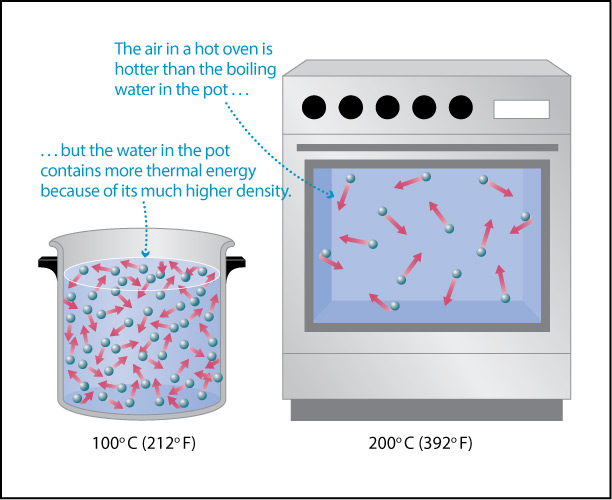
“Useful” Energy (Energy Transfer)
Objects generally contain energy in more than one form, and in any particular situation, only some forms are “useful” in the sense of being available for transfer to other objects or for conversion into some other form.
As a simple example, imagine you have a piece of popcorn and you toss it up and let it fall into your mouth. Figure 4.45 shows that the piece of popcorn has energy in several different forms, including:
- It has kinetic energy due to its motion as it falls into your mouth.
- It has thermal energy that depends on its temperature: If it is hot when you eat it, it contains more thermal energy that if it is cold.
- It has potential energy stored in its chemical bonds (“chemical potential energy”), which can release this potential energy when the bonds are broken or changed in particular ways.
In the context of eating the popcorn, however, we care only about the chemical potential energy that your body can make use of (through metabolic processes that break down or change the molecules of the popcorn). In other words, we can ignore the thermal energy and the kinetic energy of motion, because they don’t provide useful energy to our bodies when we eat the popcorn.
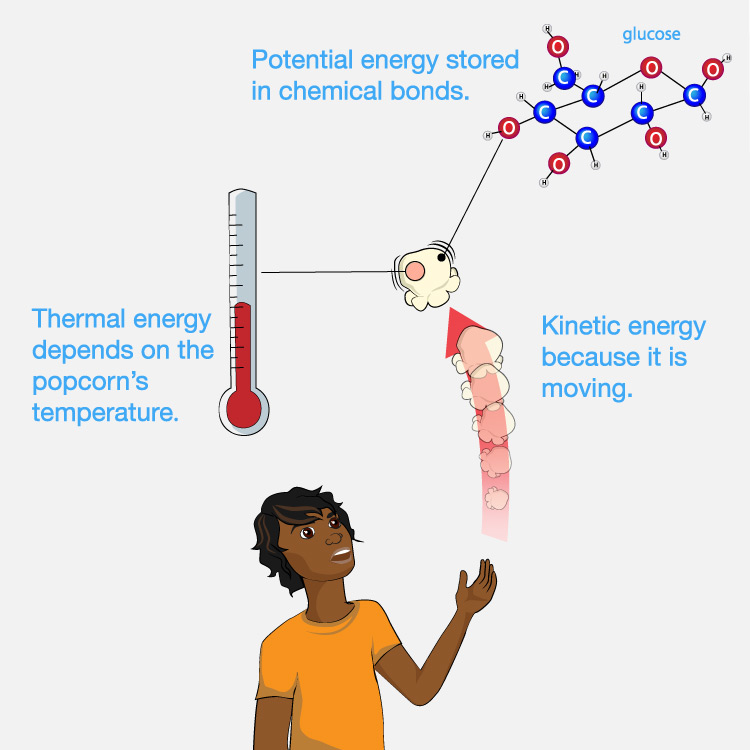
The popcorn example illustrates the idea that when we discuss energy in science, there are usually multiple types of energy involved, but we focus only on the type of energy that is “useful” in a particular context. For example:
- For any kind of food, the “useful” energy is the chemical potential energy available to our bodies.
- For objects perched on a ledge where they might fall down, or particles coming together through gravity to form a planet, the “useful” energy is the gravitational potential energy that that can be released as they fall (see this “I Was Wondering” box).
- For engineers trying to learn how to make cars safer in the event of a car crash, the “useful” energy in this context is the kinetic energy of the moving car (and its passengers).
For our current purposes, we need to understand what kind of energy is “useful” in the context of Earth system science.
- In the case of the solar energy reaching Earth, it is almost all “useful” in the sense that it could in principle be absorbed by the ground, oceans, or atmosphere. (However, some of the sunlight is reflected back to space, so this part does not actually get “used” by Earth.)
- For Earth’s internal thermal energy, the “useful” part is part that can cause movement or heating of other parts of the system. In other words, when we talk about the internal energy of Earth (or any other world), we are talking about the thermal energy (or heat) that can be transferred from one place or object to another.
Units of Energy and Power
We are now ready to discuss how we quantify energy and power in science. As with other quantities that can be measured, we need defined units of measurement, and here they are:
- For energy: The standard international unit of energy is called the joule.
- For power: The standard international unit of power is called the watt, defined as 1 joule per second:
Keep in mind that we use joules when we are talking about the total energy content of something. For example, the amount of energy we can get from food, or the amount of energy that a car can get from a liter of gasoline, or the amount of energy released by an earthquake. We use watts when we are talking about the rate at which energy is used. For example, light bulbs and electrical appliances are labeled in watts because that tells us how much energy they need for each second they are turned on.
Discussion
Using Joules and Watts Correctly
Evaluate each of the following sentences to decide if they have correctly used units of joules or watts, and explain why the usage is correct or incorrect.
- Our home refrigerator requires 200 watts of power.
- Our home refrigerator requires 200 joules of energy per second.
- Our home refrigerator requires 200 joules of energy.
- Our school’s rooftop solar system can generate a maximum of 20,000 watts of power.
- Our school’s rooftop solar system can generate a maximum of 20,000 joules of energy.
- Burning 1 kilogram of coal releases 35 million joules of energy.
- Burning 1 kilogram of coal releases 35 million watts of power.
- Our family car needs 30 million joules of energy to make the trip from our house to my uncle’s house.
- Our family car needs 30 million watts of power to make the trip from our house to my uncle’s house.
This discussion aims to give students practice at correctly interpreting and distinguishing units of energy in joules and power in watts.
- The statement uses watts correctly, since it correctly states that they are a unit of power.
- This statement is also correct because it is equivalent to the one above, simply substituting “watts” with the equivalent “joules per second.”
- This statement does not use joules correctly, because it does not make sense to state a total energy use without giving a time frame in this context.
Note: These first three statements should help clarify the general ideas, so be sure students understand them before doing the rest. - This statement uses watts correctly as a unit of power.
Note: The 20,000 watts is more commonly referred to as a 20 kilowatt system. It is the maximum power because it is what the system will generate in direct sunshine during summer. - This statement does not use joules correctly, because it does not give a time scale and therefore does not make sense in this context.
- This statement uses joules correctly in describing the total energy content of the fuel. Note: The number given is correct for the average energy content of 1 kg of coal.
- This statement does not use watts correctly, because it does not have any time or rate involved.
- This statement uses joules correctly in describing the total energy required for the drive. Note: One liter of gasoline yields about 34 million joules of energy, so assuming average driving conditions, this drive involved a distance just slightly less than what the car would get from 1 liter of gas.
- This statement does not use watts correctly, because it does not have any time or rate involved.
We’ll talk about both energy and power in our Earth science discussions, but when we are doing quantitative comparisons, we’ll more commonly talk about them in terms of power. Moreover, because we will often discuss large amounts of power, we’ll often modify watts with one of the following common metric prefixes:
- Kilo means 1,000, so a kilowatt is 1,000 watts, or 1,000 joules of energy per second.
- Mega means 1 million, so a megawatt is 1 million watts, or 1 million joules of energy per second.
- Giga (both g’s pronounced as hard g’s as in “gift”) means 1 billion, so a gigawatt is 1 billion watts, or 1 billion joules of energy per second.
- Tera means 1 trillion, so a megawatt is 1 trillion watts, or 1 trillion joules of energy per second.
For example, total world power consumption is approximately 15 terawatts, meaning that as a civilization, we use about 15 trillion joules of energy each second. As we’ll see in later chapters, we can use a number like this to evaluate how different energy sources, such as wind or solar, might be useful to us in the future.
Activity
Local Power Plant
Find out where the nearest power plant to your home or school is located, then answer these questions about it.
- How much power is the power plant capable of producing at maximum output?
- What kind of fuel does the power plant use? (For example, coal, natural gas, wind, nuclear.)
- A typical household in the United States requires an average of about 1 kilowatt of power. How many households could the power plant supply (on average)?
- Do you think the amount of power needed locally varies? If so, what do you think might cause an increase or decrease in local power demand?
This activity requires finding some data about a local power plant. Before you set students loose on this one, be sure that you confirm for yourself that the needed data are easily accessible online. If not, you may need to find it yourself first (if necessary, by calling someone at the local power plant who would know the answers) and provide the basic data to the students. Notes:
- Questions 1 and 2 should have clear answers that you can find.
- For (3): The math here should be easy for students, as long as they remember the meanings of the metric prefixes. For example, a 10 megawatt power plant is equivalent to 10,000 kilowatts, which means it could supply power to 10,000 homes (assuming 1 kilowatt per home).
- For (4): Answers will vary locally. For example, in places where air conditioning is used in the summer, the power demand will usually be greatest on hot summer days. If your area sometimes endures demand-driven blackouts, you might help students understand what kinds of power demands tend to be responsible for these.