We have talked about a number of important “big picture” concepts for our planet, but we have only touched on how we know these things. For example, you know that we learn about Earth’s interior with seismic waves, and about the geological time scale through careful study of rocks and fossils. But there are many other ways that scientists study our planet. Before you read on, try the following discussion.
Group Discussion
Studying Planet Earth
Working in small groups, try to make a list of at least 5 ways that scientists can learn about the Earth. The come together as a class and consolidate your lists into a larger list for the whole class.
The goal of this discussion is for students to think about many ways of studying Earth before we discuss some of them below. Some students may read ahead, in which case their lists may show these types of study – that’s OK, since this is just to get them thinking. As with many similar discussions earlier in the book, we ask students first to work in small groups to make a list of at least 5 ways to study Earth, then to come together as a class to combine the lists. You should use judgment in making sure that items worded slightly differently still make only one entry on the class list.
You’ve probably come up with quite a few different ways by which scientists study the Earth. The full list is very long, and new ways of studying Earth are frequently developed. Here, we’ll discuss just a few of the most important methods of study — methods that we’ll refer to again later as we study Earth in more detail.
Geological Studies
When we speak about “geological” features of the Earth, we generally mean features that we can see on the surface landscape (including the ocean floor) and features of Earth’s interior. We’ve already discussed how we learn about Earth’s interior, including the important role of seismic waves. For the surface landscape, our primary tools are careful study of rocks and fossils, which we’ll discuss further in Chapter 5.
In recent decades, one more tool has become particularly important to studying Earth’s geology: the Global Positioning System , or GPS. You are probably familiar with GPS, because it is what your phone or car uses to navigate from one place to another. GPS is also used by ships and airplanes.
The Global Positioning System gets its name from the fact that it allows anyone on Earth to determine their position — that is latitude, longitude, and altitude — very precisely. In most cases, GPS is precise to within a few meters. For example, a car navigation system will know what road you are on, because there is probably not another road within a few meters of you, though it will not know exactly which lane you are in.
Activity
GPS Accuracy
Note: This activity requires that you have smart phones with map apps. Work in groups of 2 to 3 students.
Step 1: Start by standing near each other, and open a map app (such as Google maps or Apple maps) that uses GPS to find your position. Do your phones correctly show that you are all in the same place? If not, why might that be the case?
Step 2: Start walking apart from one another. How far do you need to be apart before your phones clearly show that you have separated? Note: Because you probably won’t be able to see each other’s phones clearly as you walk apart, you may need to take screenshots after you separate, then come back together to compare to see if the phones recognized your separation; repeat this until the separation is clear.
Step 3: Discuss what you have learned about the accuracy of the GPS readings that you get from your phone. Are your results consistent with the range of accuracy of a few meters that we expect for GPS?
This optional activity will help students realize that they use GPS all the time on their phones, and gives them a chance to explore its general accuracy. Notes:
- The basic idea is for students to see that GPS is accurate to within a few meters by noticing that they must separate by more than that before their maps will all agree they are separated.
- Note, however, that phone maps often show data that would not perfectly match what a true GPS device would show (for a variety of reasons). Therefore, students might note some discrepancies. For example, their phones may initially show slightly different positions, even when they are all standing together. Use this as an opportunity for discussion about how reliable you should consider your position data to be.
- Be sure to note that, as discussed below, scientists have figured out ways to greatly enhance the accuracy of GPS for geological measurements, but these methods don’t work with the standard GPS functionality of our phones.
For geology, the importance of GPS arises from the fact that scientists have figured out ways to use it to get even more precise positions — in some cases, positions precise to only about a millimeter. This means that scientists can use GPS to track subtle movements of Earth’s surface, such as those that occur during earthquakes or volcanic eruptions. Scientists even use GPS to track the movement of Earth’s tectonic plates, despite the fact that these plates typically move at a pace that wouldn’t be noticeable to our eyes for thousands or millions of years.
The details of how GPS works are beyond the scope of what we’ll discuss here, but in case you are curious, Figure 4.25 provides a visual summary. The “system” in the Global Positioning System is a set of at least 24 satellites (there are 31 as of 2020) orbiting Earth. Their orbits are designed so that from any point on Earth, it is possible to “see” — or more accurately, receive a radio signal from — at least 3 GPS satellites at a time. This allows a GPS receiver to calculate a 3-dimensional position on Earth (latitude, longitude, altitude). Note that the satellites orbit at an altitude of a little over 20,000 kilometers, which makes them medium-altitude satellites.
Figure 4.25 (above) This educational poster provided by the U.S. government summarizes how the Global Positioning System works. Credit: NOAA, U.S. Air Force. (right) This animation shows how multiple GPS satellites are always visible (meaning that a radio signal can be received from them) from any particular location, even though Earth is rotating and the satellites are all orbiting. The location on Earth in this case is Golden, Colorado (USA). The red lines show the satellites visible at this location at a particular moment. Notice that different satellites are visible for this location at different times. As long as 3 or more satellites are visible, it is possible to measure a precise location. Credit: Paulsava.
Local Measurements (“Ground Truth” Data)
Besides learning about geological features, Earth scientists also want to learn about the atmosphere, oceans, weather, distribution of life, and much more. There are two basic ways to do this:
- We can make direct, local observations or measurements; that is, measuring something where you are located. These types of direct observations or measurements are sometimes called ground truth data , because they represent facts (“truth”) observed or measured on the ground where the observer is standing.
- We can make observations or measurements of things that are at some distance from our location through what is called
remote sensing ; in this context, “remote” means that the thing we are studying is not in the same place that we are located, but instead is “remote” from us.
Let’s first consider local (ground truth) data. There are many different kinds of local data that scientists may collect. In fact, these data are often collected even by non-scientist volunteers, including school children. For example, weather data — such as temperature, pressure, humidity, rainfall, wind speed and direction, and more — are collected by both scientists and nonscientists around the world (Figure 4.26). This type of “citizen science” (science done by nonscientists) enables scientists to have a lot more data than they might have otherwise, and more data almost always leads to more understanding.
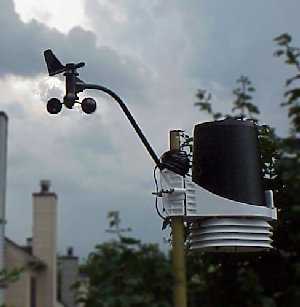
Remote Sensing
Look back at Figure 4.12, which shows Marie Tharp’s mapping of the ocean floor. Given that the ocean floor is hidden from view and that explorers in deep-sea submersibles have visited only a few locations on the ocean floor, how was she able to make such a map? The answer is that she used data obtained through the remote sensing technique called sonar (originally an abbreviation for “sound navigation and ranging”), in which sound waves are sent outward and then recorded as they bounce back (Figure 4.27).
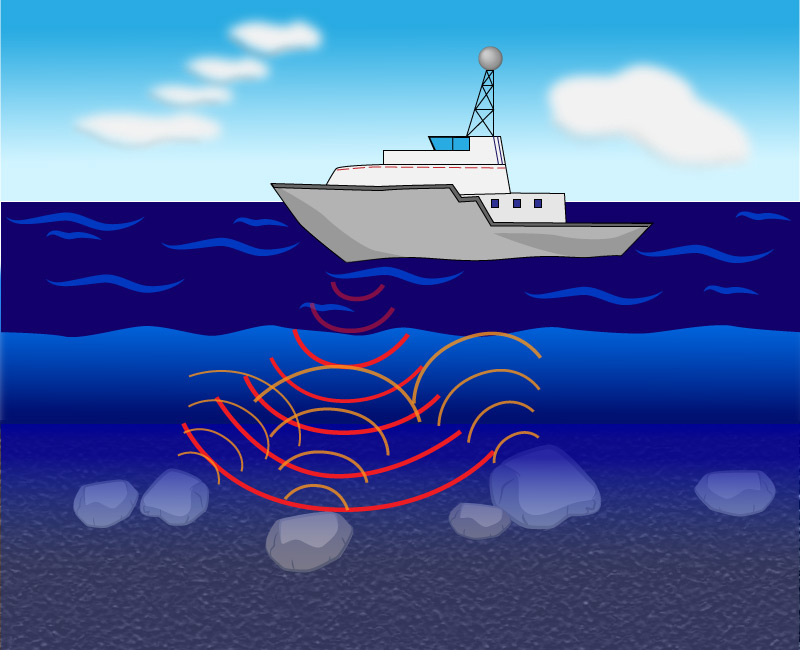
Try this question before you continue. Click to make sure you have found and understand the answer.
- In what sense is sonar a form of “remote” sensing?
Discussion
Another Word for Sonar
Sonar is sometimes also called “echolocation.” Briefly discuss why sonar is essentially the same thing as “locating an echo.” Do you know of any animals that make use of echolocation?
This very brief discussion should help students understand the concept behind sonar. In brief, an echo is simply a sound bouncing off some distant (or “remote”) surface, which is exactly the same thing that scientists do with sonar. There are many animals that use echolocation, with the best-known being dolphins and bats.
Sonar is considered a form of what is known as active remote sensing, because we must actively send out the sound waves that then bounce back to provide us with information. Two other forms of active remote sensing that you have probably heard of and that are commonly used are:
- Radar (originally an abbreviation for “radio detection and ranging”) works similarly to sonar, except instead of bouncing sound waves of distant (“remote”) objects, it bounces radio waves off them.
- Lidar (originally an abbreviation for “light detection and ranging”) works like radar except using laser light rather than radio waves.
For learning about the Earth, radar and lidar are usually used from aircraft, balloons, drones, or satellites.
Some forms of remote sensing are considered passive, since we simply observe light or sound from objects at a distant. These include taking photographs of Earth’s surface or studying the spectra of light from the surface. In fact, while sonar, radar, and lidar are all very useful, most remote sensing is passive.
Claim-Evidence-Reasoning Activity
Satellite Remote Sensing
Use evidence and reasoning to support or refute the following claim:
Claim: Scientists can use radar and lidar to conduct remote sensing observations of Earth from satellites, but they cannot use sonar from satellites.
This is a simple CER activity designed to help students begin to understand the difference between sonar, radar, and lidar. The claim is true. For their evidence and reasoning, students should realize that light, including radio waves, can travel through space, but sound cannot. Therefore it is possible to have radar and lidar instruments aboard satellites, but sonar would be useless since sound waves cannot be transmitted from space to Earth.
Wow Factor
Learning from Gravity
Coming soon . . .