At the beginning of this section, we listed six key things that gravity does. In the rest of this section, we’ll discuss how gravity does these things. We’ll start here with the first two, except we’ll go in the opposite order of how we listed them earlier:
- Gravity makes objects fall when dropped.
- Gravity holds us to the ground and gives us weight.
Note that we will only use Newton’s theory of gravity, as embodied in his universal law of gravitation. In case you are wondering why we don’t use Einstein’s theory instead, it is because both theories give equivalent explanations for all the things we will discuss here, and in that case it is easier to use Newton’s theory.
Falling Objects
Think about how an object drops when it falls (on Earth). At the moment you drop it, it is barely moving, but the farther it falls, the faster it goes. In other words, as long as air resistance doesn’t get in the way, dropped objects accelerate as they fall downward, with their speed getting faster and faster until they hit the ground. As Galileo discovered, all dropped objects accelerate downward at the same rate, which we call the acceleration of gravity.
Newton’s universal law of gravitation explains why gravity makes objects fall in this way. As a simple example, think about dropping a ball and try to answer the following questions in the context of this law, which is repeated here for reference.
Key Concepts: The Universal Law of Gravitation
In statement form:
- Every mass attracts every other mass through the force called gravity.
- The strength of the gravitational force attracting any two objects is directly proportional to the product of their masses. For example, doubling the mass of one object doubles the force of gravity between the two objects.
- The strength of gravity between two objects decreases with the square of the distance between their centers. We therefore say that the gravitational force follows an inverse square law with distance. For example, doubling the distance between two objects weakens the force of gravity by a factor of 22 = 4.
In equation form:
where Fg is the force of gravitational attraction, M1 and M2 are the masses of the two objects, and d is the distance between their centers (Figure 3.21). The symbol G represents a special number called the gravitational constant. (Its numerical value has been measured to be
G =6.67 x 10-11 m3/(kg x s2)
- For a falling ball, what are the two masses that are attracting each other through gravity?
- Given that the two objects are attracting each other, why do we notice the ball falling toward Earth rather than Earth falling toward the ball?
- In what direction does the ball fall toward Earth?
- Why does the ball accelerate, getting faster as it falls?
- If you wanted to calculate the amount of the acceleration, you would need to know the distance (d) between their centers. What is that distance in this case?
Because the objects are the ball and the Earth, the distance between their centers is the distance from the center of the ball to the center of the Earth, which is the radius of the Earth plus the height from which we drop the ball. Because Earth’s radius is so big compared to the height from which we drop a ball, we can approximate this just as the radius of the Earth, which is about 6,400 kilometers.
To summarize, falling objects on Earth accelerate downward because of the way gravity attracts them toward the center of the Earth (Figure 3.24). Note that the same thing would happen on any other world, except that the strength of gravity differs on other worlds, and a different strength of gravity will make the acceleration different.
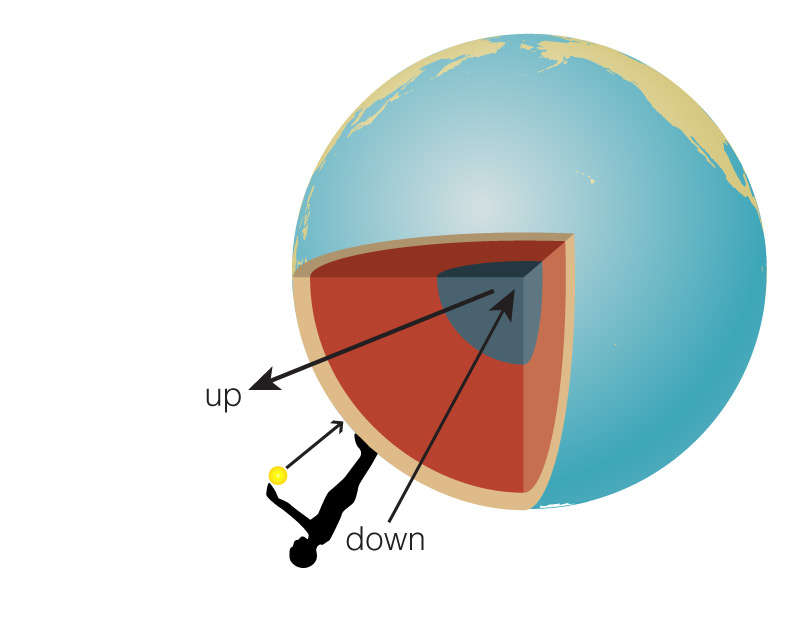
Holding Us to the Ground and Giving Us Weight
It’s fairly obvious that gravity holds us to the ground, and you can now understand why by thinking about what you already know about falling objects:
- Imagine that a hole suddenly opened up beneath you. Because gravity is pulling you toward the center of the Earth, you would immediately begin falling downward toward Earth’s center.
- Therefore, the reason you are not currently falling toward Earth’s center is because the solid ground is stopping you. In essence, the ground is pushing back against gravity, preventing you from falling.
- To summarize, gravity is always trying to pull you downward, so when the ground (or a table or floor or other solid object) is stopping you, you will feel yourself pressed against it.
The amount of the force that you feel as you are pressed against the ground is what we call your weight. In other words, weight is simply what you feel as a result of gravity holding you to the ground (Figure 3.25).
Activity
Weight on a Scale
Find a small bathroom scale and place on it a heavy object that has a handle. (For example, a small suitcase filled with books.)
- How much does the object weigh?
- Push down (just a little – not too hard) on the object while it is still on the scale. Does the weight shown on the scale get smaller, larger, or stay the same? Why?
- Now pull up on the object’s handle a little (but not so much as to pull it off the scale). How does the weight on the scale change now? Why?
- What can you conclude about what a scale actually measures?
This simple activity is designed to help students understand that a scale is actually measuring how hard something is pressed against it, which will help set the stage for them to understand weightlessness next. For the specific questions:
1. They should record the weight of their object.
2. Pushing down makes the scale read higher, indicating that the object is now pushing down harder on the scale. This occurs because the object is now pressing down on the scale with the force of its own weight due to gravity plus the additional force of your push.
3. Pulling up makes the scale read lighter, indicating less of a push against the scale. This occurs because the object is now pressing down on the scale with the force of its own weight due to gravity minus the force of your upward pull.
4. The scale measures the net force (a concept that should be familiar from physical science) with which the object is pressing against it, which is the force due to gravity (weight) plus (or minus) any other force that is also acting on it.
Mass and Weight
Based on what you’ve just learned, you probably now realize that there’s a subtle but important distinction between what we call “mass” and what we call “weight,” even though we often use the terms interchangeably in daily life:
- Your mass is the amount of matter in your body.
- Your weight is the force that you feel due to gravity pressing your mass to the ground.
Your mass is therefore the same no matter where you are located – on Earth, in space, on the Moon, or anywhere else. In contrast, the weight you feel will vary. For example, because gravity is weaker on the Moon, you will weigh less on the Moon, even though your mass would still be the same as on Earth.
Freefall and Weightlessness
Think about videos of astronauts floating weightlessly in space. You can see that they are weightless, because they are not pressed against any ground or the spaceship walls. Another way you can tell they are weightless is that they can do all kinds of tumbles and twists, which you can’t do with your feet stuck to the ground. So the astronauts are obviously weightless. But have you ever been weightless?
Weightlessness in Space
Video. This video shows some of the tricks that astronauts can do when floating weightlessly in space. Credit: NASA/International Space Station.
You might be surprised to learn that the answer is yes. To see why, imagine that you are standing on a scale on the edge of a diving platform. The scale reads your weight which, as you already know, tells you how hard gravity is making you push against it. Now suppose that someone pushes both you and the scale off the platform, so you both start falling toward the water. If you could look at the scale as you fall, what would it read?
Before you continue, turn to a neighbor and discuss what you think the scale will read while you and the scale are both falling toward the water.
You’ve probably realized that, because you are no longer being pressed against the scale, the scale will read zero as you fall (Figure 3.26). This is not an illusion. The fact that the scale cannot measure any weight for you during your fall means that, just like an astronaut in space, you are weightless during your fall. As further evidence of this fact, notice that if you were a skilled diver, during your fall you could do tumbles and twists just like astronauts do while floating weightlessly in space.
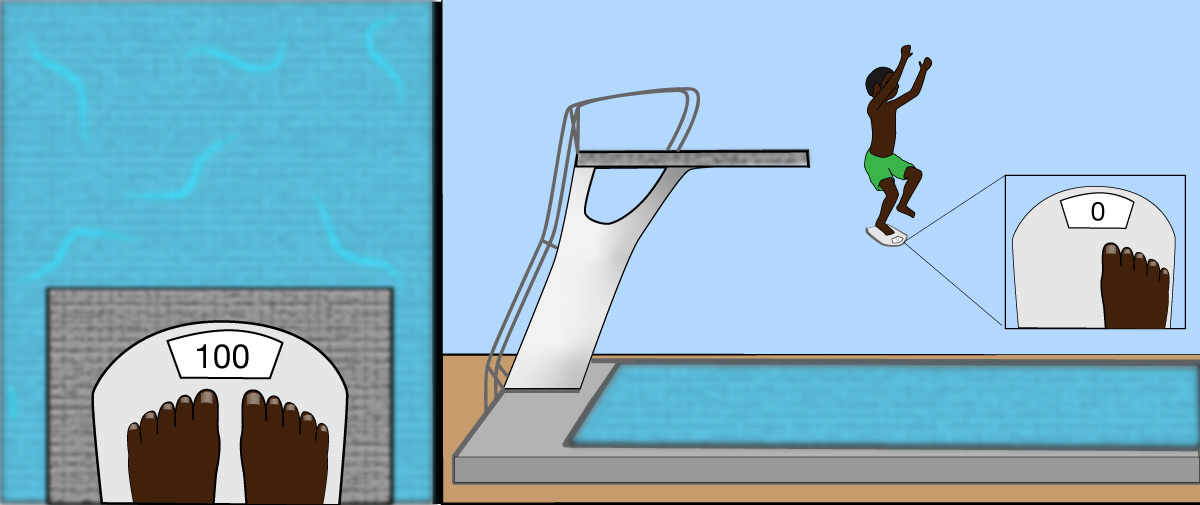
More generally, we say that you are in freefall whenever there is no ground or anything else for you to push against, even if you are going up (like when you jump up on a trampoline) rather than down. During freefall, you will always be weightless (as long as there is no air other resistance to slow you down). This means you can become weightless right now if you wish, simply by jumping off a chair. Of course, your weightlessness will last only from the time your feet leave the chair until they hit the ground.
Weightlessness in Space
This brings us to a very common misconception, which concerns the question of why astronauts are weightless in space.
Claim-Evidence-Reasoning Activity
“No Gravity in Space” – True or False?
You’ve probably heard people say that astronauts are weightless in space because there’s “no gravity in space.” But is this true? Working in small groups, use evidence and reasoning to decide whether the following claim is true or false:
Hint: To help your thinking, also consider this question: What keeps the Moon in orbit of Earth, or Earth in orbit of the Sun?
The claim is false. The given hint should be very helpful. Students should already know that gravity holds the Moon in orbit of Earth and Earth in orbit of the Sun, which makes it quite obvious that there is gravity in space. In fact, gravity explains why the space station orbits the Earth (rather than flying off into deep space).
Once they recognize there is gravity in space, they will be prepared to continue on and learn the real reason for weightlessness which, as explained in the discussion and slideshow 3.27 that follow, is that orbit represents a continual state of freefall, and you are always weightless during freefall.
To understand the real reason that astronauts are weightless in space, it helps to think about what it takes to get into space in the first place. Imagine that you had a really tall tower, as shown in Slide Show Figure 3.27. Step through the slide show, and watch what happens as you leave the tower at different speeds.
Slide Show Figure 3.27 - This set of illustrations explains why astronauts are weightless and float freely in space. If you were launched from a tall tower with enough speed, you would travel forward so fast that you’d be in orbit of Earth. Above the atmosphere, where there is no friction to slow you down, you could continue to orbit indefinitely.
To summarize, the real reason that astronauts are weightless when orbiting Earth is because they are always “falling” toward Earth, even though they never hit the ground. In other words, they are in a constant state of freefall, and as we’ve discussed, you are always weightless in freefall.
In case you want another fun way to think about this: In the book The Hitchhiker’s Guide to the Galaxy, author Douglas Adams wrote that the trick to flying is to “throw yourself at the ground and miss.” In essence, this is exactly what orbiting spacecraft do. Like the person shot out of the cannon in the slide show, rockets are launched at such high speed that even though they have essentially been “thrown at the ground,” they are going so fast that they always “miss.”
Claim-Evidence-Reasoning Activity
Astronauts in Freefall
Based on what you have learned so far, work in small groups to use evidence and reasoning to support the following claim:
The claim is true. Combining what they have learned from Figure 3.26 and slideshow 3.27, students should recognize that: (1) they are weightless when jumping off a chair because they are in freefall, and (2) astronauts are weightless because they are in constant freefall when orbiting the Earth.
Note that, while we’ve talked only about the situation of astronauts orbiting Earth, the same idea applies to astronauts anywhere in space. As long as no engines are firing to create a force that astronauts would feel, then motion anywhere in space is due only to gravity and is therefore essentially free fall. For example, imagine you are in a spacecraft with engines off on the way from Earth to Mars. Once you escape from the region where Earth’s gravity can hold you in orbit, your spaceship will be “freefalling” due to the Sun’s gravity. As you approach Mars, its gravity will become noticeable, and if you fire your engines briefly to enter Mars orbit, you will then be in freefall around Mars. To summarize, you are in freefall — and therefore weightless — whenever your motion is governed only by gravity.
In fact, strange as it may sound, the same idea applies to spaceships and even to entire worlds. For example, the International Space Station would weigh more than 400 tons if it were on the ground, but because it is orbiting in space, it weighs nothing at all, which is why it floats in space as it orbits. As for worlds, try the next discussion to consider Earth.
Claim-Evidence-Reasoning Activity
Earth is Weightless!
Earth has an enormous mass, which explains why it has strong enough gravity to hold us to the ground and to hold the Moon in orbit. But does Earth weigh anything? Based on what you have learned in this section, work in small groups to use evidence and reasoning to support the following claim:
The claim is true. Students should be able to recognize this by direct analogy to what they’ve learned about astronauts being weightless. Just as astronauts on the Space Station are in freefall about Earth, Earth is similarly in freefall as it orbits the Sun. Using the weightlessness of astronauts as evidence, students can argue that if Earth is in freefall as it orbits the Sun, then it too must be weightless. This discussion should also help students think further about the distinction between mass and weight.