Let’s pick back up with Ptolemy’s Earth-centered model (see Figure 3.4) in about 150 A.D. It was tedious and complicated, but it seemed to work, at least within the accuracy of the naked eye measurements that were possible at the time. As a result, there wasn’t much reason to question it. But that changed over time, for two major reasons.
- First, errors in the model tended to add up over time, so its predictions gradually got worse.
- Second and perhaps more importantly, instruments used to measure planetary positions in the sky got much better over time, and this made the errors more noticeable (Figure 3.6).
As a result, by the 1400s and 1500s, it was becoming quite obvious that Ptolemy’s Earth-centered model simply did not work very well. And if the model didn’t work, then it couldn’t be correct. The stage was set for a rethinking of Earth’s place in the universe.
Activity
Observations Versus Explanations
As you will see, the discovery that Earth is a planet going around the Sun required coming up with good explanations for observations of planetary positions in the sky. This means it is very important to understand the difference between an observation (meaning something we can see or measure) and an explanation (an attempt to describe the reason why something we observed has occurred).
-
- Consider the following statements that might be heard in everyday life. Working in small groups, decide whether each statement is an observation or an explanation, and explain why.
- James is wearing a yellow shirt.
- James wears a yellow shirt when his other shirts are dirty.
- The defendant's DNA was found at the crime scene
- The defendant only intended to scare the victim.
- The defendant's calendar says he was at lunch when the crime occurred.
- Plastic melts when it reaches a temperature that breaks bonds that hold its structure intact.
- The plastic melted when I held it near the light bulb.
- Continuing in the same small groups, now consider a set of statements related to astronomy. Again, decide whether each statement is an observation or an explanation, and explain why.
- Stars can shine brightly for millions to billions of years.
- Stars shine with energy generated by nuclear fusion in their cores.
- The universe is expanding because it began with a Big Bang.
- More distant galaxies are moving away from us faster than nearby galaxies.
- The orderly orbits of planets arose from their formation in a rotating gas cloud.
- All planets orbit the Sun in the same direction and nearly the same plane.
- The Andromeda Galaxy is similar in structure to our own Milky Way.
- The Andromeda and Milky Way galaxies formed in the same way.
- Consider the following statements that might be heard in everyday life. Working in small groups, decide whether each statement is an observation or an explanation, and explain why.
- Come together as a class and compare the results from each group. Did all the groups come up with the same answers about which statements are observations and which are explanations? If not, discuss until you come to a class consensus on each statement.
As the intro to the activity indicates, it is very important to be able to distinguish between statements that represent observations versus statements that make attempts at explanation. Perhaps surprisingly, this can be difficult for many students. This activity should help students understand the difference. Note that this activity could be done at most any point in the rest of this chapter. We’ve suggested doing it here before delving into the details of the Copernican revolution, so that students will all be clear on the meaning of “observations.”
Answer are as follows:
For #1:
- James is wearing a yellow shirt. Observation.
- James wears a yellow shirt when his other shirts are dirty. Explanation (because it is explaining observations of when James wears various color shirts).
- The defendant’s DNA was found at the crime scene. Observation, since this was found and the DNA presumably identified through observational/measurement techniques.
- The defendant only intended to scare the victim. Explanation, since you can’t observe a state of mind or motive.
- The defendant’s calendar says he was at lunch when the crime occurred. Observation; note that this one is subtle, because the fact that the calendar says this doesn’t mean it actually happened. But the fact that the calendar has this entry is an observation.
- Plastic melts when it reaches a temperature that breaks bonds that hold its structure intact. Explanation, suggesting a molecular basis for the conditions under which the melting occurs.
- The plastic melted when I held it near the light bulb. Observation, since it states something that you saw happen.
For #2:
- Stars can shine brightly for millions to billions of years. Observation; for example, the Sun is a star and we know that it is billions of years old.
- Stars shine with energy generated by nuclear fusion in their cores. Explanation: We cannot see into the cores of stars, so we cannot directly observe how they produce energy. We can only infer it from theory.
- The universe is expanding because it began with a Big Bang. Explanation: the expansion is observed, but the “because” makes this an explanatory statement about that observation.
- More distant galaxies are moving away from us faster than nearby galaxies. Observation: We measure this fact, which is how we know the universe is expanding.
- The orderly orbits of planets arose from their formation in a rotating gas cloud. Explanation of why we observe patterns in planetary orbits.
- All planets orbit the Sun in the same direction and nearly the same plane. Observation, because today we can directly measure planetary orbits.
- The Andromeda Galaxy is similar in structure to our own Milky Way. Observation of galaxy structure.
- The Andromeda and Milky Way galaxies formed in the same way. Explanation of why both galaxies have similar structures.
Copernicus
The big change began with a Polish scientist named Nicolaus Copernicus (1473–1543). Copernicus was not the first person to suggest that Earth orbits the Sun; recall that this idea had been proposed by Aristarchus some 1,800 years earlier [Section 3.1.2]. Indeed, in his writings, Copernicus acknowledged that he was aware of Aristarchus’s proposal.
Why did Copernicus decide to try a Sun-centered model? If you think back to what we’ve discussed, you’ll realize that there were almost certainly two main reasons:
- Ptolemy’s Earth-centered model wasn’t working well, and therefore needed to be replaced by something.
- Copernicus was surely aware that the apparent retrograde motion of the planets in the sky has a much more natural explanation in a Sun-centered model (see Figure 2.34) than in an Earth-centered model (see Figure 3.4). Although his writings do not directly state how this fact influenced him, it is very likely that Copernicus made the decision to try a Sun-centered model because it seemed so much simpler and more natural than an Earth-centered model.
Once he started down the path of using a Sun-centered model, Copernicus spent a lot of time working out its mathematical details so that he could use it to predict planetary positions. In that process, he also discovered that he could use the geometry of his model to calculate how long it took each planet to orbit the Sun and how far (compared to Earth) each planet was from the Sun. The fact that his model led to a mathematically simple layout for the solar system gave him added confidence that he was on the right track in putting the Sun at the center.
Fyi, Copernicus was able to calculate only relative planetary distances; that is, their distances in units of Earth’s orbital distance (the astronomical unit, or AU, discussed in Chapter 1). The same was true for other astronomers of the era, including Kepler and even Newton. Measuring the value of the AU in absolute units, such as miles or kilometers, did not become possible until the 1800s.
Copernicus ultimately wrote a book describing his model in depth (Figure 3.7). The book was titled De Revolutionibus Orbium Coelestium, which translates to “On the Revolutions of the Heavenly Spheres.” It was published in 1543 — the same year in which Copernicus died; in fact, he apparently received the first printed copy of his book on the very day that he died.
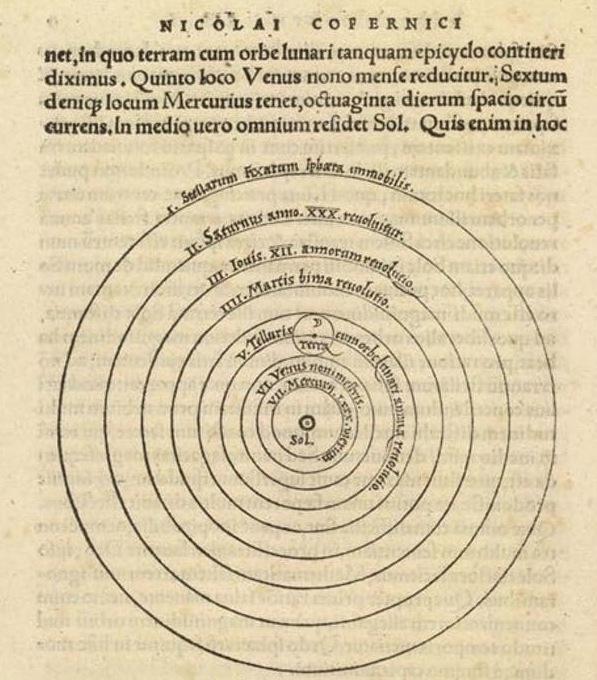
Copernicus’s book spread quickly among European scholars and scientists, drawing wide interest. It did not immediately convince everyone, for reasons we’ll discuss next. However, the fact that Copernicus began the process with a book title that included the word “revolution” explains why we refer to the dramatic change that overturned the Earth-centered view of the universe as the Copernican revolution.
Discussion
The Meaning of “Revolution”
Discuss the following questions in small groups; after you discuss each one, you can open the answers for each and see how your group’s thoughts compare.
- The word “revolution” is a noun that can be used with two fairly distinct meanings. What are they? Which meaning of the word did Copernicus intend when he used the word “revolutions” in his book title (“On the Revolutions of the Heavenly Spheres”)?
The two meanings are:
- “Revolution” can refer to one celestial body going around another, such as the revolution of Earth around the Sun.
- “Revolution” can be a sudden or radical change, such as the changes that occurred with the American revolution or the industrial revolution.
Copernicus clearly had the first meaning in mind.
- There are two different verbs that go with the two meanings of the noun “revolution.” Can you figure out what the verbs are?
- All three words (“revolution” and the two related verbs) have the same Latin root: revolvere, which literally translates as “to roll back.” Does this Latin root seem to make sense for either or both of the definitions of “revolution”?
The idea of “rolling back” makes sense for “revolution” in terms of celestial motion, because an orbiting object keeps returning to the same point with each orbit. However, it is difficult to see how the Latin root applies to a sudden or radical change, particularly since “revolutions” are usually seen as starting something new, not returning to something old.
- Historically, no one knows for sure how the word “revolution” obtained its second meaning. However, there is no clear evidence that the word was ever used in that sense until after Copernicus published his book. Do you think that Copernicus’s book might have inspired the second meaning? If so, how?
This is a matter of opinion, but it certainly seems possible. Copernicus’s book led to a sudden and radical change in human thinking about the universe, from the idea that Earth was at the center to Earth being just one of the planets orbiting the Sun. Perhaps this is how the word came to mean a sudden or radical change more generally. If so, the “Copernican revolution” is even more aptly named!
This discussion offers a great opportunity to show how science and language may be intertwined. Note that while a number of people have claimed that (as in question 4) Copernicus’s book was indeed the reason that “revolution” acquired its second meaning, the evidence for this appears to be circumstantial, so no one really knows if this is the correct origin story.
Why Copernicus’s Idea Was Not Immediately Accepted
To understand why Copernicus’s Sun-centered model was not immediately accepted, you have to remember that scientific models must be able to make predictions that can be tested. In this case, the key predictions were those for planetary positions in the sky.
You might guess that Copernicus’s model would have made much better predictions than Ptolemy’s. After all, Copernicus had the right idea in putting the Sun at the center, while Ptolemy used the wrong idea of Earth at the center. But it didn’t.
I was wondering...
Did anyone else consider a Sun-centered model before Copernicus?
What went wrong? The answer is that while Copernicus had been willing to overturn the ancient idea of an Earth-centered universe, he still believed that “heavenly perfection” required that all heavenly motion had to occur in perfect circles. Because planetary orbits are not perfect circles (they are ellipses, as we’ll discuss shortly), his model made inaccurate predictions. He tried to improve the predictions of his model by adding additional complexity to it (including using “circles upon circles” like those in Ptolemy’s model), but it still didn’t work very well.
In the end, Copernicus’s complete model was no more accurate and no less complex than Ptolemy’s model. Given that fact, few people were willing to throw out thousands of years of traditional belief in an Earth-centered universe when the new model worked just as poorly as the old one.
Nevertheless, many scholars and scientists of the time liked Copernicus’s idea. We don’t know for sure what drew these people to the Sun-centered model, but its much simpler explanation for apparent retrograde motion probably played a major role. Copernicus himself had died, but others picked up where he left off.
Activity
Debate the Copernican Model
Imagine that you are students in the year 1560. Your teacher (in the year 1560) has taught you about Ptolemy’s Earth-centered model, explaining that it is the generally accepted model of the universe. But your teacher has also introduced you to this “newfangled” idea from Copernicus, claiming that Earth actually orbits the Sun.
Based on what you have learned so far, hold a class debate about Ptolemy’s model versus the Copernican model. To do this, divide your class into three groups:
- Group 1 will go first, defending the claim that planets orbit the Sun.
- Group 2 will go next, defending the claim that planets and the Sun orbit the Earth.
- Group 3 will then consider the evidence and reasoning presented by each of Groups 1 and 2, and vote on which team presented the strongest case.
Your teacher will serve as the judge, making sure that all groups present and consider only evidence that was available as of the year 1560.
Note: Students in Groups 1 and 2 should take some time to come to agreement within the group on which pieces of evidence support their case most strongly, and in preparing to explain how the evidence supports their argument. Students in Group 3 should discuss the evidence presented before rendering a group verdict on the debate winner.
As described within it, this activity is essentially a mock trial in which you’ll select two groups of students from your class to represent two legal teams, one on each side of the debate (one for Ptolemy’s model and one for Copernicus’s), and the rest of the class will serve as the jury. You will serve as judge to keep the debate on track. Be sure that you steer students to sticking with what was known in the year 1560, as discussed in the text above.
Data and Modeling
In very general terms, doing science always requires two things: data and modeling. The data come from observations and experiments. The models seek to explain why we find that data that we do. We test the models by comparing their predictions to the data.
After Copernicus published his book in 1543, scholars and scientists were faced with a key problem: Neither the old (Ptolemy’s) nor the new (Copernican) models agreed well with the data (observed planetary positions). It was therefore obvious to them that they needed a better model. They also recognized a need for better data against which to test any new model. The problem was that all existing data had been obtained by naked eye using hand-held instruments, and this did not provide enough precision for careful tests of how model predictions compared to actual planetary positions.
Key Concepts: Data and Modeling
In science, we use models to try to explain a set of observations or experiments. This means that we need accurate data (from observations and experiments) in order to determine which models may be correct and which ones are wrong.
Two people ended up playing particularly important roles in developing better data and better models: a man named Tycho Brahe provided the data, and Johannes Kepler provided the models. We’ll talk about them in a moment, but first we need to talk about angle measurement so that you’ll be able to appreciate their work.
Arcminutes and Arcseconds
You are already familiar with angle measurement in degrees, which is defined by the fact that there are 360° in a full circle. Sometimes, however, we need to measure angles that are much smaller than 1°, and for that we divide degrees into smaller units as shown in Figure 3.8:
- We subdivide each degree into 60 arcminutes (symbolized by ‘ ).
- Then we further subdivide each arcminute into 60 arcseconds (symbolized by ” ).
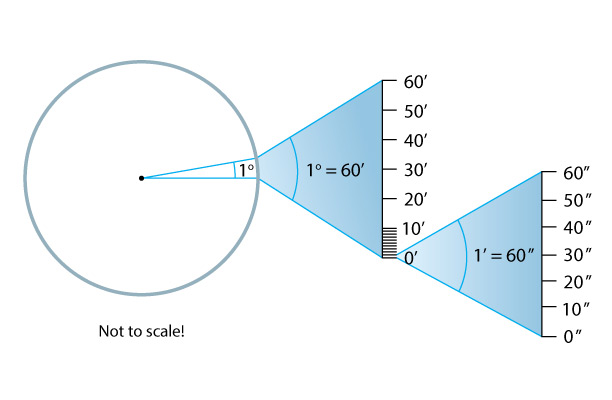
In other words, 1 arcminute (1') is 1/60 of 1° and 1 arcsecond (1") is 1/60 of 1', or 1/3600 of 1°. Notice that these are very small angles. Recall that the width of a single finger held at arm’s length is about 1° (see Figure 2.8). This means that one arcminute is less than the thickness of your fingernail at arm’s length, and one arcsecond is considerably less than the thickness of a human hair at that distance.
Quiz
Note: As always, after you finish the quiz, be sure you read the feedback that explains the correct answers, to make sure you understand the reasons for these answers.
Tycho’s Data
Tycho Brahe (1546–1601), usually known simply as Tycho (pronounced “tie-koe”), was an eccentric Danish nobleman. When he was 20 years old, he lost much of his nose in a sword fight with another student over who was the better mathematician. He then spent the rest of his life wearing a replacement nose that he made himself out of silver and gold.
Tycho recognized the need for better observations. The telescope still had not yet been invented, so Tycho set out to find a way to make more precise observations by naked eye. He developed numerous new instruments and built an observatory that worked much like a giant protractor (Figure 3.9).
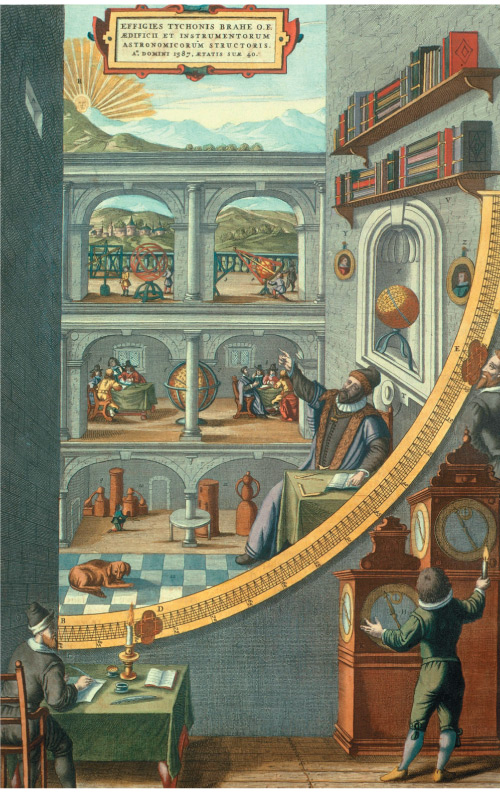
Tycho’s instruments and observatory allowed him to make naked-eye measurements of the positions of planets and stars in the sky that were accurate to within about 1 arcminute — far better than anyone had ever before achieved. Tycho and his assistants made observations for more than two decades, thereby acquiring the data that would finally prove the Earth-centered model to be wrong.
Wow Factor
Parallax Strikes Again!
Tycho was convinced that his data held the key to coming up with a correct model for the heavens, but he himself struggled to come up with one. He therefore sought help. In the year 1600, he hired a man named Johannes Kepler (1571-1630) for this purpose. As Tycho lay dying just over a year later, he handed over all his data to Kepler. Kepler later wrote that while Tycho lay on his death bed, he kept repeating over and over the words “Let me not seem to have lived in vain.”
Discussion
The Meaning of Tycho’s Life, Part 1
Consider Tycho’s statement above: “Let me not seem to have lived in vain.” What do you think he meant by this, and why do you think he said it to Kepler? Discuss in small groups, and we will return to this question after we discuss Kepler.
This brief discussion is intended to make sure students spend at least a couple minutes thinking about the story of the Copernican revolution to this point. Notes:
-
- This is the first part of a short 2-part discussion, with the second part coming at the end of the discussion below about Kepler.
- For this question: It is generally assumed that Tycho was imploring Kepler to make good use of his data, with the implication that the data represented his life’s work, and that his life would have been “lived in vain” unless it proved useful.
Kepler’s Model
When Kepler began work on creating a new model, he shared Copernicus’s beliefs that the model should be Sun-centered and that it should use only perfect circles for planetary orbits. After years of effort, he succeeded in creating a model with perfect circles that matched most of Tycho’s observations quite well. The match was not perfect, but even in the worst cases (which were for the planet Mars), this model with circular orbits predicted planetary positions that were within 8 arcminutes of Tycho’s observed positions. This is quite close, since 8 arcminutes is barely one-fourth the angular diameter of the full moon.
Kepler surely was tempted to attribute such small discrepancies to errors by Tycho. But he was convinced that Tycho’s observations had been made and recorded very carefully. He therefore concluded that it must be the circular orbits of his model, not Tycho’s data, that were wrong. About this fact, Kepler wrote:
If I had believed that we could ignore these eight arcminutes, I would have patched up my hypothesis accordingly. But, since it was not permissible to ignore, those eight arcminutes pointed the road to a complete reformation in astronomy.
With his decision to abandon perfect circles, Kepler began to try other shapes for orbits, and ultimately found the correct answer: Earth and other planets do indeed orbit the Sun, but their orbits take the shapes of the special ovals known as ellipses . Moreover, Kepler found that planets move faster in the portions of their elliptical orbits in which they are close to the Sun and slower when they are farther away (Figure 3.10).
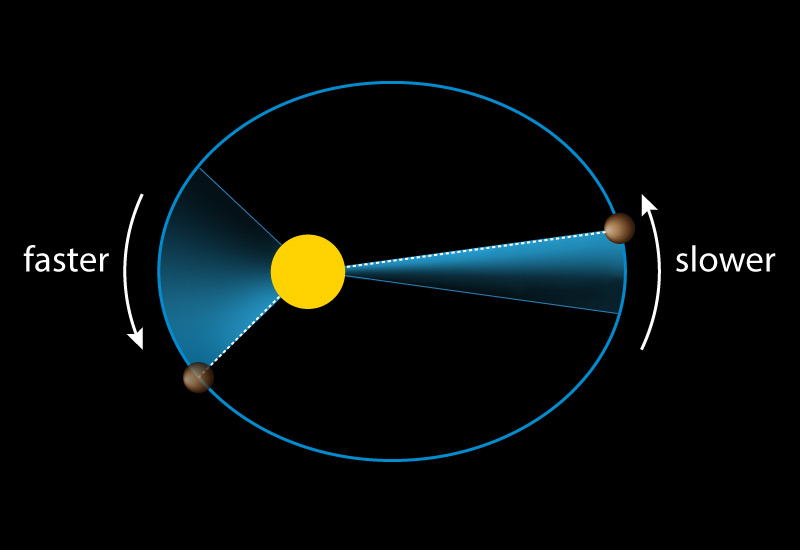
Activity
Draw an ellipse
Kepler’s major breakthrough came when he adopted elliptical rather than circular orbits for planets going around the Sun. In this activity, you will see how ellipses compare to circles by drawing both shapes.
Materials:
- a board or cardboard on which you can place tacks and they will remain in place
- two tacks
- pencil
- a piece of string that you can tie around a pencil or tie in a loop
Draw a circle.
Step 1: Tie one end of a string to a pencil and tack the other end of the string to a board or a piece of cardboard.
Step 2: Pull the string tight and drag the pencil around the tack, as shown in Figure 1.
This should make a circle, because your pencil will have traced a path that is the same distance from the tack at all points. This distance is called the radius of the circle, and the tack is at the center of the circle.
Draw an ellipse.
Step 1: Place both tacks on the board, separated by a few centimeters.
Step 2: Loop the string around the pencil and both tacks, as shown in Figure 2. With the pencil looped into the string, drag it around the tacks to draw an ellipse. Note that the positions of the tacks are called the foci (singular, focus) of the ellipse. Kepler discovered that planetary orbits are ellipses with the Sun at the location of one focus (and nothing located at the other focus).
Step 3: Move the two tacks slightly farther apart, then draw another ellipse. How does this ellipse compare to the first one?
Step 4: Move the two tacks closer together, then draw another ellipse. Does this ellipse look more like a circle or less like a circle then your earlier ellipses?
Step 5: Move the tacks even closer together, and again draw a new ellipse. Based on your work, use evidence and reasoning to support the following claim: A circle is a special ellipse in which both foci are at the same point (that is, the distance between the foci is zero).
This activity simply has students use string and tacks and pencil to draw a circle and then to draw ellipses of different eccentricity. For the questions asked in Steps 3 to 5 of the ellipse drawing: Students should notice that the ellipse gets more elongated as the foci move apart, and more circular as the foci come together. This should allow them to recognize that a circle is simply a special case of an ellipse, in which both foci are at the same point (the center).
Journal Entry
Trusting the Data
Put yourself in Kepler’s place when he discovered the discrepancies of up to 8 arcminutes with Tycho’s data. That is, assume that you believe deeply that “heavenly perfection” requires that orbits be perfect circles, but you also trust that Tycho worked carefully in collecting his data. You therefore have a choice: You can stick with your belief in circular orbits if you assume that Tycho just made a few small errors, or you can decide that your deeply held belief in circular orbits must be wrong. Which choice do you think that you would have made in that situation? Write a paragraph or two in which you state the choice you would have made and explain why.
Teacher Notes: This journal entry is intended to get students to think about how they personally would deal with a situation like Kepler faced. There is no correct answer, and in fact, the answer is not obvious. After all, until he found a different shape that worked, it’s likely that even Kepler continued to wonder if Tycho’s data might have been in error. (He wrote the words quoted above after he had landed on a model that worked.) So any grading of this entry should be based on how well students articulate the rationale behind the choice they make. For example, some students may argue that you should always trust the data (but make sure they give a reason why), while others might argue that beliefs should be considered equally valid until proven otherwise.
Kepler summarized his discoveries with three precisely stated mathematical laws that we now call Kepler’s laws of planetary motion . He published the first two of these laws, which were the ones needed to match Tycho’s data, in the year 1609. (The third law, which he published a decade later, describes how the time it takes each planet to orbit the Sun — called the orbital period — depends on its distance from the Sun.)
Kepler’s laws represent a model of planetary motion that can be used to predict the locations of planets in our sky at any time. Just as with the earlier models of Ptolemy and Copernicus, the process works basically like this:
- Start by observing the current positions of planets in the sky.
- Then use the mathematics of the model to predict future (or past) locations for the planets.
The critical difference is that while the models of both Ptolemy and Copernicus made noticeably inaccurate predictions, Kepler’s model gave essentially perfect predictions. Kepler’s model also had a second important advantage over the earlier ones: It was much simpler. Recall that calculations with Ptolemy’s model were extremely tedious and complex, and the same was true for Copernicus’s model with the complexity he was forced to add in his attempt to keep circular orbits.
In contrast, Kepler’s model is so simple that it is possible to build clockwork-like mechanisms that can reproduce planetary motion. Figure 3.11 shows one such device, in which each planet moves along an elliptical track, driven by clockwork built to follow Kepler’s laws. Of course, today it is much easier to program Kepler’s laws into a computer than to build a mechanical device. This is essentially how modern software can make perfect predictions of past and future planetary positions.
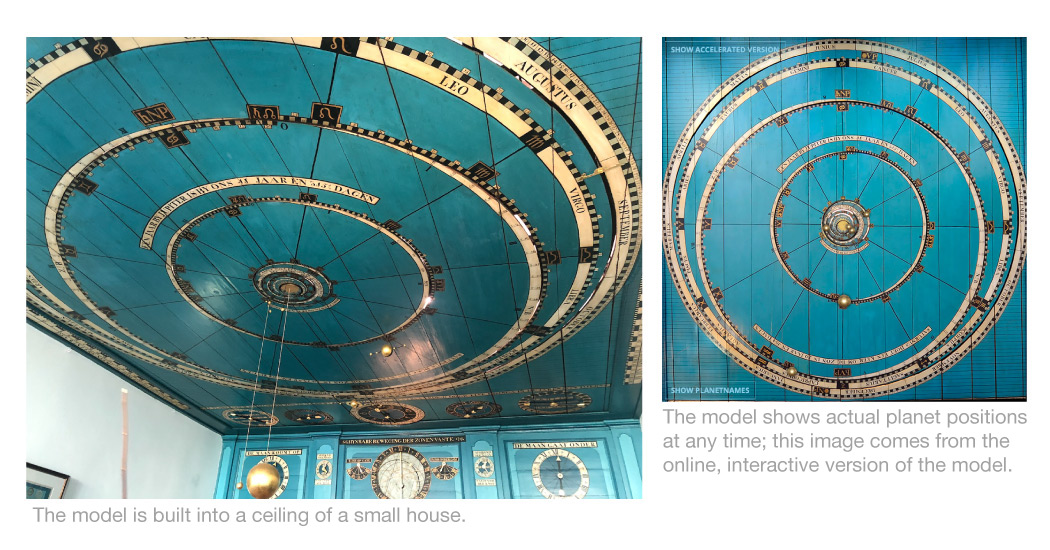
Discussion
The Meaning of Tycho’s Life, Part 2
Resume the short discussion you had earlier about Tycho’s statement, “Let me not seem to have lived in vain.” Suppose that Tycho had never hired or met Kepler, so that his data would never have been put to work in finding the correct laws of planetary motion. Do you think that would have meant that Tycho’s life had been “lived in vain?” What lessons might we draw from the collaboration of Tycho and Kepler?
This resumes the earlier discussion. Notes:
- The first part of this question is opinion-based, and students may differ in their opinions as to whether Tycho’s life would have been “lived in vain” if Kepler had not succeeded in making good use of his data.
- For the second part of the question, we encourage you to help students see how the story of Tycho and Kepler shows the value of the collaboration, both in general and in this specific case.
Do the Math
Eccentricity: How close is close enough?
Coming soon