The fact that all of Earth’s systems are dynamic means that there must be sources of energy to drive all that action. Where do you think that energy comes from? To help you answer that question, we’ll start by looking at two examples, each representing a simple model of a type of change that occurs on Earth.
Two Brief Examples
We are briefly introducing the rock and water cycles here in order to help students understand the role of energy in driving Earth system processes. Note, however, that you should not expect students to understand them in any detail just yet. We’ll do that in Chapters 5 (for the rock cycle) and 6 (for the water cycle). Here, you should expect students to view the cycles only an intuitive way, based on what they can infer from the figures of the cycles alone. An added benefit of this approach is that when we cover the cycles in more depth in the coming chapters, students will already have some familiarity with them.
The two models we will consider are called the rock cycle and the water cycle. We will study each of these in more depth later (the rock cycle in Chapter 5 and the water cycle in Chapter 6), along with other, similar models. Here, we introduce them only briefly, so that you can begin to think about the energy sources that drive them.
The Rock Cycle
The rock cycle is a model that describes the general ways in which rocks can change from one form to another. You might (or might not) be surprised to learn that rocks can change form over time, but if you think about it, you’ll realize that you were already aware of this fact. For example, think about a volcano: A volcanic eruption sends out rivers of hot, molten rock; this molten rock then cools and solidifies into solid rock (Figure 4.37).

Rock that forms after molten rock solidifies is called igneous rock . The rock that is forming in Figure 4.37 is igneous because it is solidifying from molten lava. (Molten rock can also solidify underground, and is still called igneous in that case.)
Figure 4.38 shows the basic rock cycle. Notice that it has igneous rock at the top, and shows that this rock can in some cases be transformed into two other general categories of rock, called metamorphic rock and sedimentary rock . We’ll discuss these rock types and how the form in more detail later, but you can already understand the basic ideas just by studying the figure. Use the quiz below to make sure you understand how to read the diagram.
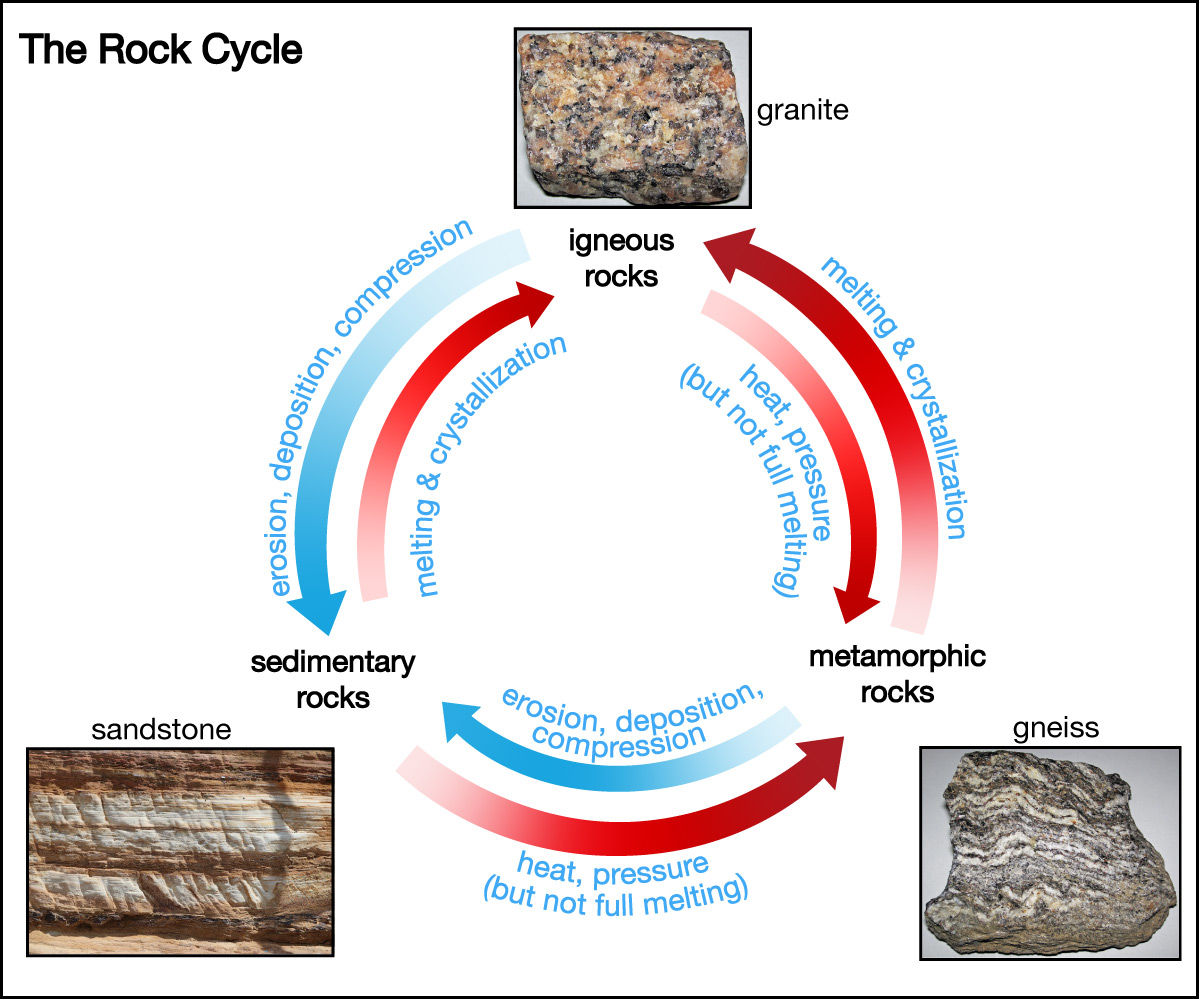
Quiz – The Rock Cycle
Answer the following questions about the rock cycle by studying Figure 4.38.
Note: As always, after you finish the quiz, be sure you read the feedback that explains the correct answers, to make sure you understand the reasons for these answers.
The rock cycle operates on long time scales — typically millions of years. You can understand why by considering what is involved in the processes shown on the arrows in Figure 4.38:
- The red arrows represent transformations of rocks that require melting or substantial heat and pressure. These processes can occur only deep beneath Earth’s surface, generally in the upper mantle. Therefore, for any surface rock to undergo these processes, it first has to wait for the action of plate tectonics to carry it down into the mantle, and this takes millions of years.
- The blue arrows represent transformations in which erosion breaks rocks apart, and pieces that end up deposited in a particular place (deposition) then become compressed into sedimentary rock. As you might imagine, compressing rocks until they become cemented together in a new form takes a very long time — again, millions of years.
Claim-Evidence-Reasoning Activity
A Rock Cycle on the Moon?
Based on what you know about the Moon, use evidence and reasoning to support or refute the following claim.
Claim: The Moon does not have a rock cycle.
The claim is true: There is no rock cycle on the Moon. Any of the following would could serve as evidence that students might cite to support this claim:
- The rock cycle includes erosion, and erosion requires wind or rain, neither of which are present on the Moon. Therefore, the Moon cannot have a rock cycle.
- The Moon’s craters indicate an ancient surface, which means rocks are not being changed on the Moon like they are on Earth. This also tells us that there is no rock cycle on the Moon.
- The Moon does not have plate tectonics, and as the above bullets point out, without plate tectonics there is no way to cycle rocks into the interior for heating or high pressure.
Note that we have not yet covered any of the above facts about the Moon (lack of erosion, ancient surface, lack of plate tectonics) specifically in this text, but through collaborative effort it is likely that most students will be able to recognize at least one of these ideas and therefore correctly support the claim.
The Water Cycle
Our second sample model is the water cycle , shown in Figure 4.39. This cycle represents the way in which water is “recycled” from the oceans to the atmosphere, to rivers and streams, and back to the oceans. Study the cycle, then try to answer the questions that follow to help you think about the cycle and the time scales involved.
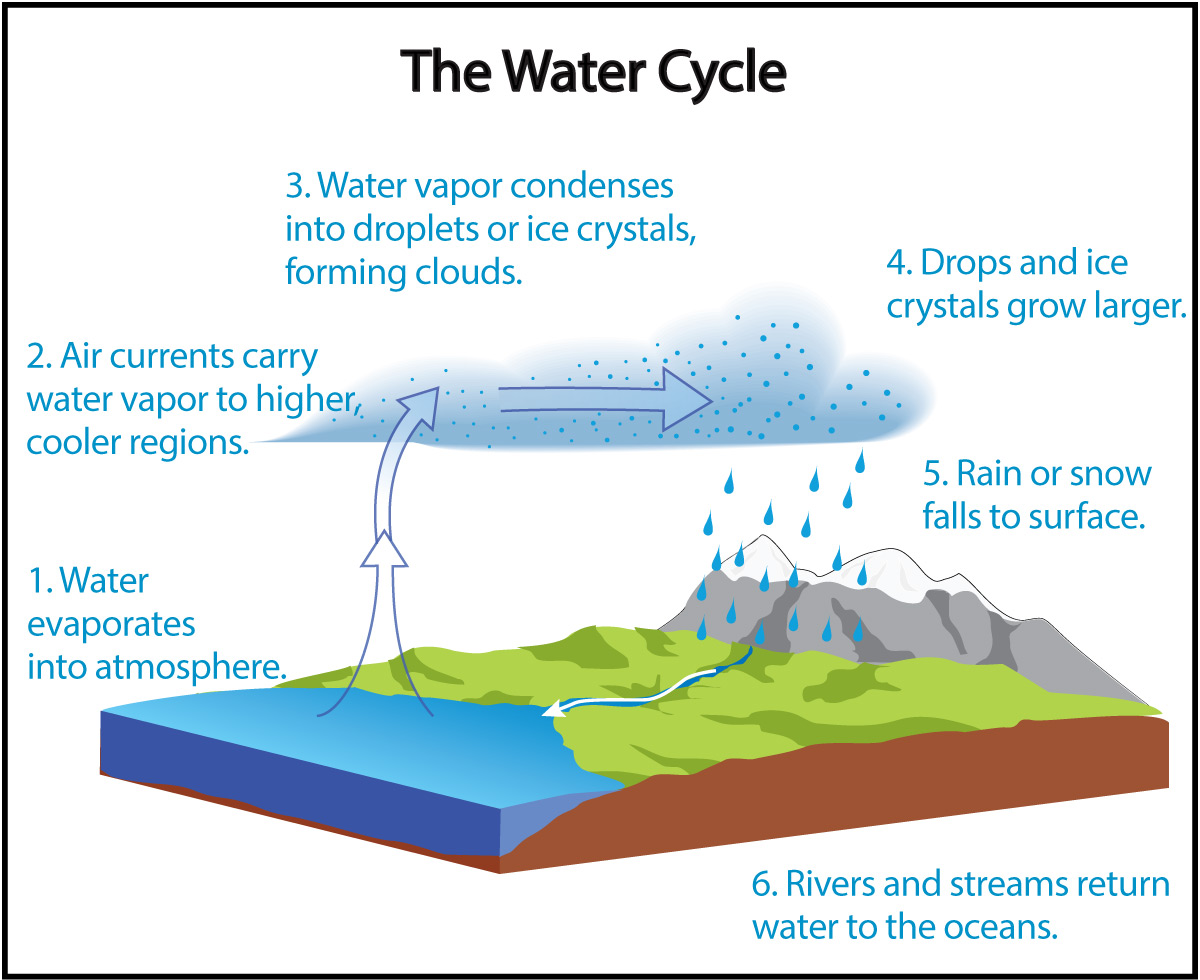
Discuss the following questions with a classmate. Then click to open the answers to see if they agree with what you came up with.
- Which two steps in Figure 4.39 do you think happen in the shortest amounts of time? (Consider only the six labeled steps; that is, don’t count the time that water actually spends in the ocean before evaporating.)
Steps 1 and 5 happen the most rapidly. Step 1, evaporation, is always occurring, and once a particular water molecule escapes from the ocean surface, it enters the atmosphere immediately. Step 5, which is rainfall or snowfall, also happens rapidly, since once rain or snow starts falling, it does not take long for it to reach the ground.
- Which step in Figure 4.39 takes the longest amount of time?
Step 6 takes the longest time, particularly if the rain or snow falls far inland, where it has a long distance to travel through streams and rivers to reach the ocean. This often take years even if the water takes a fairly direct path to the oceans. It can take much longer if the water first sinks into the ground to become groundwater or freezes at the top of a glacier.
- Multiple choice: Consider steps 2 and 3 in Figure 4.39, in which evaporated water condenses to form clouds that release the water as rain or snow. Based on what you know about weather, do you think this process typically takes: (a) a few hours; (b) a few days; (c) a few months; or (d) a few years. Explain how you know.
The answer is (b), a few days. One way to know this is by thinking about the satellite images you see in a local weather forecast. These images will typically show clouds or storms heading toward your area at least a few days before they reach your area, which is part of how forecasters are able to predict that rain or snow is coming. You know it is not much more than a few days, since weather forecasts are not reliable more than about 7 to 10 days in advance.
- The water cycle in Figure 4.39 shows the rain or snow falling over land. How would the cycle look different if the rain or snow instead fell over the ocean, and how would this affect the time required for the cycle?
The cycle would be simpler (as shown below), because the water would return directly to the oceans, rather than having to make its way back to the oceans through rivers or streams. This would also make the cycle take less time, since it removes Step 6, which is the step that takes the longest time.
This diagram shows what the water cycle looks like if the rain or snow falls directly over the ocean rather than over land. The cycle is then simpler and faster, since the water returns directly to the ocean, skipping Step 6 (from Figure 4.39), which is the step that takes the longest time.
Spheres of the Rock and Water Cycles
Notice that the rock cycle primarily involves the geosphere and the water cycle primarily involves the hydrosphere. However, as you saw earlier when you considered the roles played by each of the four spheres in an apple, there are almost always interactions between the different “spheres.” Try the following discussion to help you think about these interactions in the rock and water cycles.
Discussion
Rock and Water Cycle Interactions
Discuss the following questions in small groups or as a class.
- In what sense might you think of the rock cycle as involving only the geosphere?
- In what sense might you think of the water cycle as involving only the hydrosphere?
- Looking deeper, identify at least one way in which the rock cycle would not be possible without the water cycle. (Hint: Think about the processes listed on the arrows that point to sedimentary rocks in Figure 4.38.)
- Identify at least one way in which the water cycle would not be possible, at least in the form shown in Figure 4.39, without the geosphere.
- Going deeper, can you make a case that the atmosphere is also necessary to either or both of the rock cycle and water cycle?
- Based on what you’ve learned and what you know about other planets, could there be an Earth-like rock cycle or water cycle on any of the other planets of our inner solar system (Mercury, Venus, Mars)?
Remember that we’ll be covering these cycles in more depth in Chapters 5 and 6. Here, the goal is simply to make sure students understand the basic ideas behind these cycles and how there are almost always interactions between Earth’s four major “spheres.”
- (1) All types of rocks are part of the geosphere, so in that sense the rock cycle involves only the geosphere.
- (2) All forms of water (ice, liquid water, water vapor) are part of the hydrosphere, so in that sense the water cycle involves only the hydrosphere.
- (3) The figure shows that making sedimentary rocks requires erosion, and most erosion is caused by water.
- (4) Rivers and streams cut through the land, which is part of the geosphere. So without the geosphere, there would be no rivers and streams.
- (5) Both cycles require the atmosphere. The water cycle requires the atmosphere, because that is where water evaporates into and condenses from. Therefore, without the atmosphere, there could not be a water cycle. And because we’ve already found that the rock cycle requires the water cycle, the atmosphere is crucial to that as well.
- (6) No. Mercury lacks an atmosphere, making it like the Moon in lacking these cycles. Venus is too hot for liquid water and therefore has no water cycle or Earth-like rock cycle (though Venus may have a somewhat different rock cycle, since it almost certainly has active volcanoes that can produce new igneous rocks). Mars does not have any liquid water on its surface today and therefore also lacks a water cycle and rock cycle. However, Mars had surface water in the distant past, so it would have had a water cycle and rock cycle at that time. Note: To answer this part, students will need to remember what they learned from the virtual tour of the solar system back in Chapter 1; we will cover this again in Section 4.2.2.
Energy Drivers of the Rock and Water Cycles
We’re now ready to take a look at the sources of energy that drive the rock and water cycles. Let’s start by identifying the energy inputs that are needed for the water cycle.
Discuss the following questions with a classmate. Then click to open the answers to see if they agree with what you came up with.
- Why is an input of energy required for Step 1 in Figure 4.39 (repeated below)?
- What is the source of the energy for Step 1?
- Notice that Steps 2 and 3 both involve movement of air: Step 2 has air currents that carry water vapor upward and Step 3 has cloud movements that are driven by winds. What energy source drives these air movements?
Notice that the rest of the water cycle does not require any input of energy, since it just involves gravity causing the rain to fall downward and rivers to flow downhill . In other words, the water cycle is driven entirely by the energy that our planet receives in the form of sunlight from the Sun.
Claim-Evidence-Reasoning Activity
Energy for the Water Cycle
Based on what you’ve learned so far, use evidence and reasoning to support or refute the following claim.
Claim: The water cycle is ultimately driven by a single source of energy: the energy that Earth receives in the form of sunlight from the Sun.
The claim in this CER activity has already been established through the questions and answers above, but this idea is so important that it is worth having students think through it again with the CER format. In this case, they should essentially reconstruct the ideas above, noting that the only external energy input to the water cycle comes from the Sun.
Let’s turn now to the rock cycle, which is slightly more complex in terms of its energy inputs.
Discuss the following questions with a classmate. Then click to open the answers to see if they agree with what you came up with.
- Is solar energy (energy from the Sun) involved anywhere in the rock cycle?
- The red arrows in Figure 4.38 show processes in the rock cycle that require high temperature to melt or transform rocks. What is the source of energy for these processes?
In other words, the parts of the rock cycle that involve erosion of rocks are driven by energy from the Sun, while the parts that transform rocks through melting or high heat are driven by the energy of Earth’s internal heat. (Recall from an earlier box that this internal heat comes from a combination of energy the Earth acquired when it formed and energy that is still being released into the interior by radioactive decay.)
Summary: Energy Drivers of the Earth System
We’ve used the rock and water cycles as examples, but in fact the two sources of energy that power those cycles are also the energy sources for virtually all processes that occur in the Earth system. That is, the energy for virtually everything that happens on our planet can ultimately be traced to just two major sources:
- Energy from the Sun (solar energy).
- Energy from Earth’s own internal heat.
Generally speaking, processes that occur on the surface are driven by solar energy, while processes that occur underground, such as melting of rock under volcanoes or the motions of tectonic plates, are driven by internal heat (Figure 4.40).
Figure 4.40 – Virtually all processes that occur on Earth are driven by just two major sources of energy: energy from the Sun and energy from Earth’s own internal heat.
Claim-Evidence-Reasoning Activity
Energy of the Spheres
- Based on what you’ve learned so far, use evidence and reasoning to support the following claim.
Claim: Dynamic processes that occur in the geosphere are driven almost entirely by internal heat, while those of the hydrosphere, atmosphere, and biosphere are driven almost entirely by solar energy.
- Can you think of any exceptions to the general rule in the claim? That is, are there cases where solar energy affects the geosphere, or internal energy affects the hydrosphere, atmosphere, or biosphere? Give as many examples as you can.
This CER activity asks students to generalize ideas that we’ve already covered, then consider possible exceptions. A few key points:
- Geosphere: Dynamics of the geosphere are indeed driven primarily by internal heat. Students can cite their earlier discussion of the rock cycle, along with the internal heat driving volcanoes, earthquakes, and plate tectonics in general. Exceptions: As we’ve already discussed for the rock cycle, Earth’s surface, which is part of the geosphere, is often shaped by erosion, where solar energy plays a role. Other exceptions include processes that occur underground where water from rainfall has seeped in; since rainfall was involved, this also involves solar energy.
- Hydrosphere: As we discussed with the water cycle, the dynamics of the hydrosphere almost always are driven by solar energy. Exceptions: Exceptions occur in places where the hydrosphere is affected by the release of Earth’s internal heat, such as at underwater volcanoes or in geothermal hot springs.
- Atmosphere: Similar to the hydrosphere, nearly all atmospheric dynamics can be traced to solar energy, with the only significant exceptions being associated with volcanoes, which add gas and some small particles of dust to the atmosphere. Of particular interest: Studies have shown that the small particles can in some cases affect the weather or climate.
- Biosphere: We usually think of sunlight as the only energy input for the biosphere, since sunlight drives photosynthesis, and we usually think of all lifeforms as either growing through photosynthesis (for example, plants, algae) or eating organisms in a food chain that ultimately traces back to photosynthesis (such as animals eating plants or eating other animals that ate plants). Exceptions: Scientists now know that there are some living organisms that get their energy from Earth’s internal heat, rather than from the Sun. This is the case, for example, for some organisms that live deep underground or near deep-sea volcanic vents. This fact is very important to the search for extraterrestrial life, because it is the reason that scientists think there is a possibility of life underground on Mars or in places like the subsurface ocean of Jupiter’s moon Europa: There is no sunlight in these places, but there might be enough internal heat to make life possible.