Look once again at the global surface winds shown in the Earth Project of Figure 6.2–1, repeated again below. You’ll see many complex wind patterns, but if you look closely, and compare to winds on other dates (as you may already have done for question 6 in the Activity at the beginning of Section 6.2), you might see some general wind patterns. In this section, we’ll explore these general wind patterns, building gradually more sophisticated models to explain them.
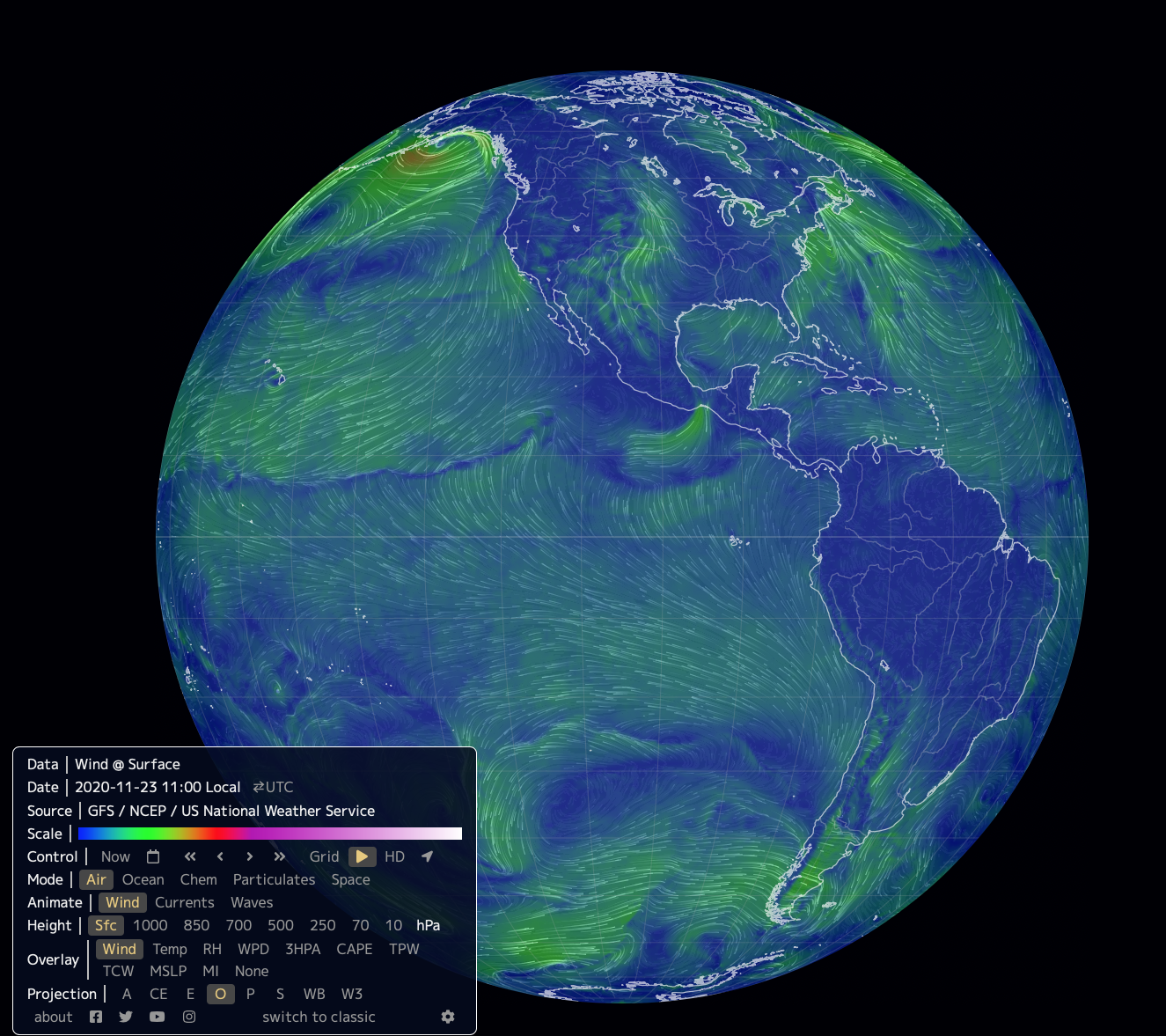
Be sure to note that, in this section, we are focusing on surface winds, meaning winds near the surface. We will not consider high-altitude winds (such as jet streams), which require more complex models.
General Surface Wind Directions
Let’s start by looking at the general directions in which Earth’s winds blow. We’ll first consider the most simplified case, in which we ask only whether the winds blow generally from the east (called easterly winds) or from the west (called westerly winds). Use the following questions to discover this simplified pattern for yourself. Notice that the wind direction depends primarily on latitude .
Discuss the following questions with a classmate as you study the Earth Project surface wind map (wind @ surface) in Figure 6.2–1. Then click to open the answers to see if they agree with what you came up with.
- Look at winds near Earth’s equator. Would you say that these winds generally blow in any particular direction? If so, what direction is it?
- Look at winds in polar regions; that is, winds that blow in regions near the north and south poles. (You may need to rotate the interactive globe in Figure 6.2–1 to see these clearly.) The wind patterns will probably be more complex than the patterns you see near the equator, which illustrates the fact that polar weather is usually more dynamic than equatorial weather. Still, if you look at the most general pattern, would you say that the winds flow in the same direction or in the opposite direction of the equatorial winds?
- Now look at winds in-between the equatorial and polar regions; that is, look at mid-latitude winds, such as those blowing over most of the Unites States. How does the general direction of these winds compare to the general directions of equatorial and polar winds?
- Challenge Question: Think about the paths you have seen for hurricanes and other tropical storms, which form near the equator, where the oceans are warm. In which general direction do these storms usually travel over the open ocean? Explain how this makes sense based on your answers to the earlier questions.
If you look at the paths of tropical storms, you will see that they generally travel from east to west. This should make sense given that these storms form near the equator (because they get their energy from warm, tropical waters). They therefore travel with the equatorial winds from east to west.
Figure 6.2.2–1 summarizes the general patterns that you should have discovered as you answered the questions above. Be sure to remember that this is highly simplified model of wind patterns, since real wind patterns are much more complex.
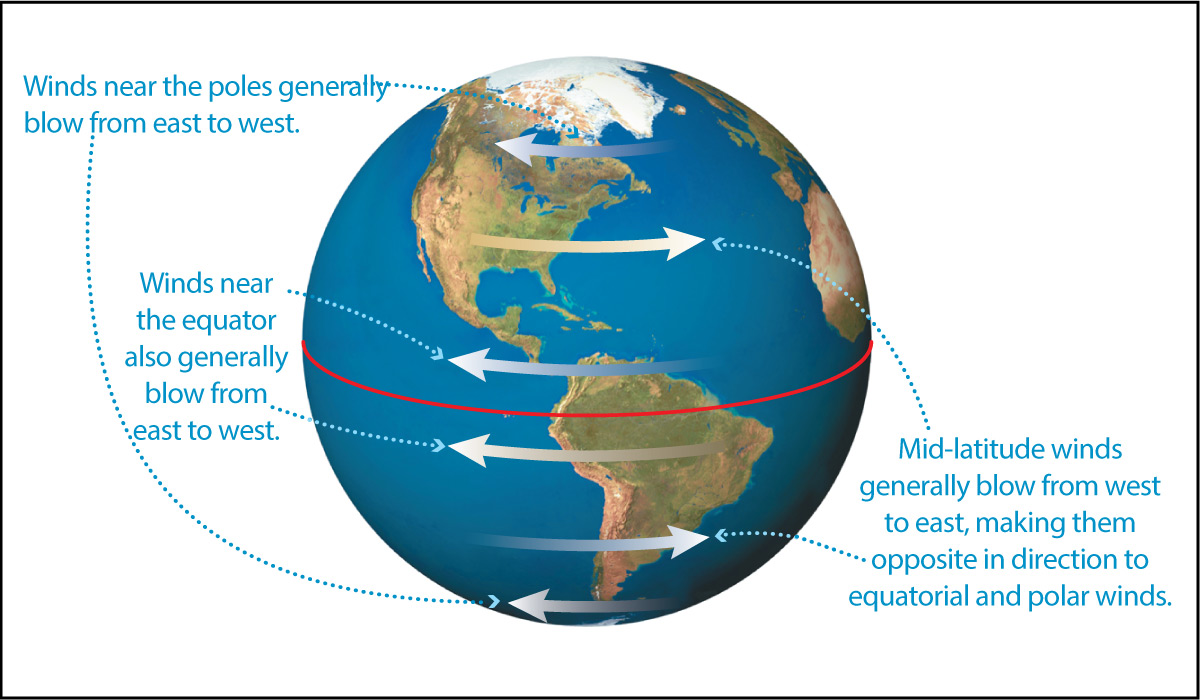
To summarize, if we ignore the complicating effects of land distribution, seasons, and changing weather, Earth has the following general, global wind pattern:
- Equatorial and polar winds blow from east to west…
- … while the mid-latitude winds in between them blow from west to east.
You are probably wondering why our planet would have these three general sets of winds (equatorial, mid-latitude, and polar). To understand the answer, we need to look a little more deeply at the effects of solar energy input and the Coriolis effect , building on what you learned about them in Section 6.2.1.
A Non-Rotating Global Circulation Model
We’ll start by building a model for Earth’s global wind patterns (also called the global circulation) that ignores Earth’s rotation. That is, we’ll start by considering only the effects of solar heating.
As you know, solar energy input is much stronger near the equator and weaker near the poles (see Figure 6.2.1–2). This energy imbalance means that heat will tend to flow from the equator to the poles. If Earth were not rotating, this heat flow would occur in a very simple way: The warm equatorial air would rise upward, then flow toward the poles, where it would sink back down.
In other words, without rotation, the fact that sunlight warms the equator more than the poles would create two large, global circulation cells in Earth’s atmosphere, one carrying heat from the equator toward the North Pole and the other carrying heat from the equator toward the South Pole. Figure 6.2.2–2 shows what this simple model would look like.
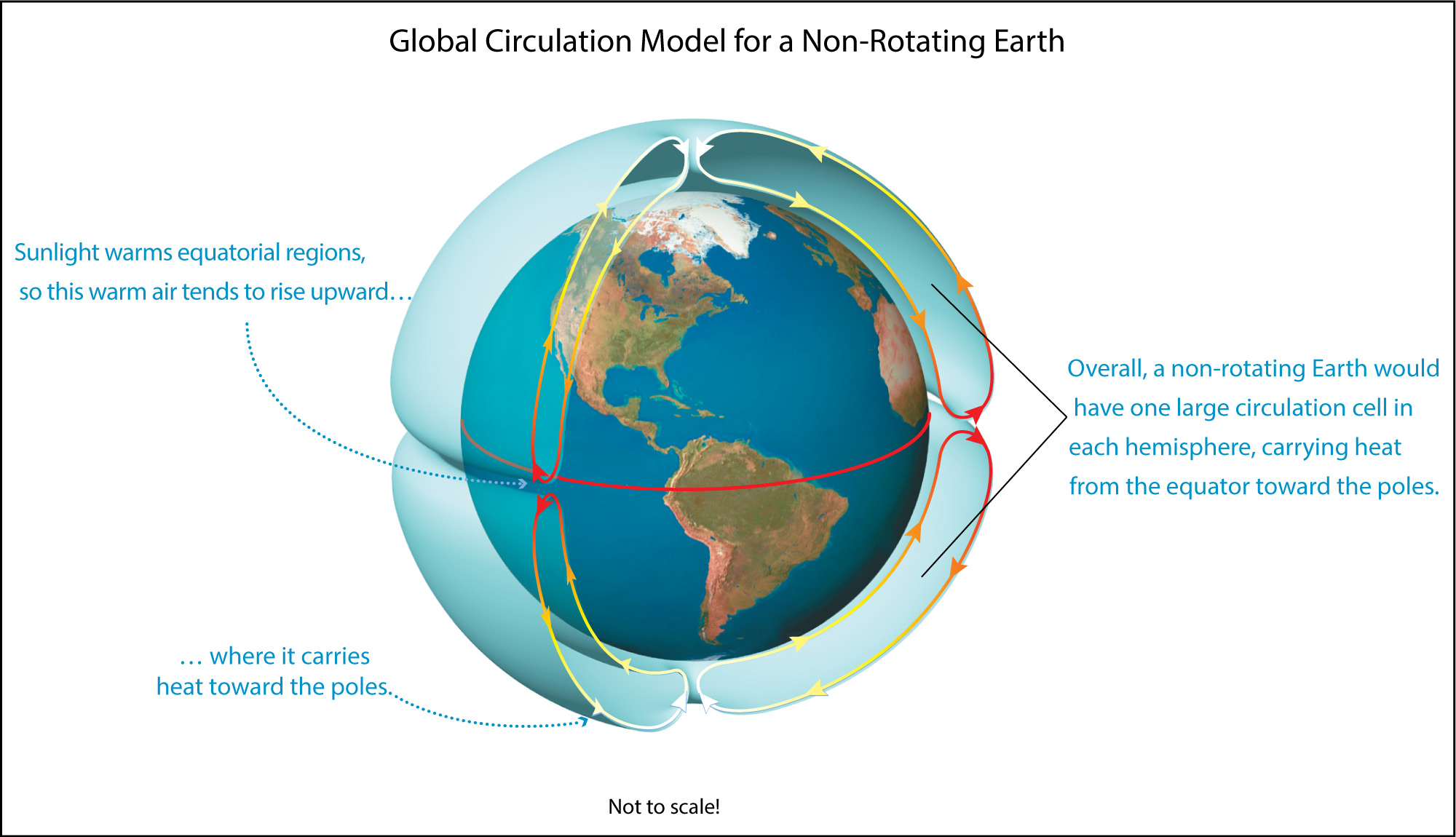
Discussion
Understanding Circulation Cells
Discuss these questions in small groups or as a class.
1. Using the ideas of thermal energy transfer that you learned about in Section 6.2.1, explain why we should expect heat to flow from the equator toward the poles.
2. How are the two global circulation cells shown in Figure 6.2.2–2 similar to convection cells like those in shown in Figure 5.3.2–4?
3. As you will see shortly, Earth’s actual circulation pattern is more complex than the simple pattern of Figure 6.2.2–2, which shows what it would look like if Earth were not rotating. However, there is one planet in our solar system that does have a simple circulation pattern like that. Which planet is it, and why? Hint: Consider planet atmospheres and rotation rates, which you can review in our virtual tour of the solar system.
This discussion checks that students understand the basic idea behind the circulation model with no rotation.
- (1) This question is essentially the same as question 2 from the Discussion “Local and Global Thermal Energy Transfer” at the end of Section 6.2.1, except now students have seen the circulation pattern. Again, the answer is that the greater sunlight strength at the equator means there is more thermal energy in equatorial regions than polar regions. Therefore, since thermal energy tends to move from regions of more heat to regions of less heat, the equatorial heat will tend to migrate toward the poles.
- (2) In both cases, the circulation pattern is driven by the fact that warm material is less dense and tends to rise, while cool material is more dense and tends to fall. The only real difference between other convection cells (in the mantle, or in storms) and these atmospheric circulation cells is scale, as the air circulation cells span the globe.
- (3) Students should recognize that for a planet to have the simple “non-rotating” circulation pattern of Figure 6.2.2–2, it needs to meet two criteria: it must have a fairly substantial atmosphere (so there is plenty of heat to circulate) and it must rotate very slowly, so it close to being non-rotating. The planet that meets these criteria is Venus. Indeed, Venus’s circulation cells are so effective at transporting heat that it has essentially no temperature difference between its equator and poles, despite the much greater strength of sunlight at its equator. Note: We will also discuss Venus in the I was Wondering box that comes shortly below.
The Three-Cell Circulation Model
The simple model of Figure 6.2.2–2 for a non-rotating Earth is a useful starting point, but it is obviously not realistic, since Earth does rotate. Recall that rotation causes the Coriolis effect, which deflects winds traveling long distances on Earth. Use the following questions to think about how the Coriolis effect will change the wind patterns of the non-rotating model.
Discuss the following questions with a classmate. Then click to open the answers to see if they agree with what you came up with.
1. Look closely at the Northern Hemisphere circulation cell shown in in Figure 6.2.2–2. Which direction are surface winds flowing in that cell?
2. Now look at the Southern Hemisphere circulation cell. Which direction are surface winds flowing in that cell?
For the Southern Hemisphere circulation cell, surface winds would be blowing northward, going from the South Pole toward the equator.
3. Consider again the Northern Hemisphere surface winds, starting from the North Pole. In which direction will these winds be deflected by the Coriolis effect? Give your answer as a direction (north, south, east, or west).
Remember that the Coriolis effect deflects winds to the right in the Northern Hemisphere. Therefore, as shown below in Figure 6.2.2–3, winds that are flowing southward from the North Pole will be deflected toward the west.
4. Now consider the Southern Hemisphere surface winds, starting from the South Pole. In which direction will these winds be deflected by the Coriolis effect? Give your answer as a direction (north, south, east, or west).
For the Southern Hemisphere, the Coriolis effect deflects winds to the left. Figure 6.2.2–3 shows that this means the winds flowing northward from the South Pole will also be deflected toward the west.
Figure 6.2.2–3 summarizes the ideas you should have come up with when answering the questions above.
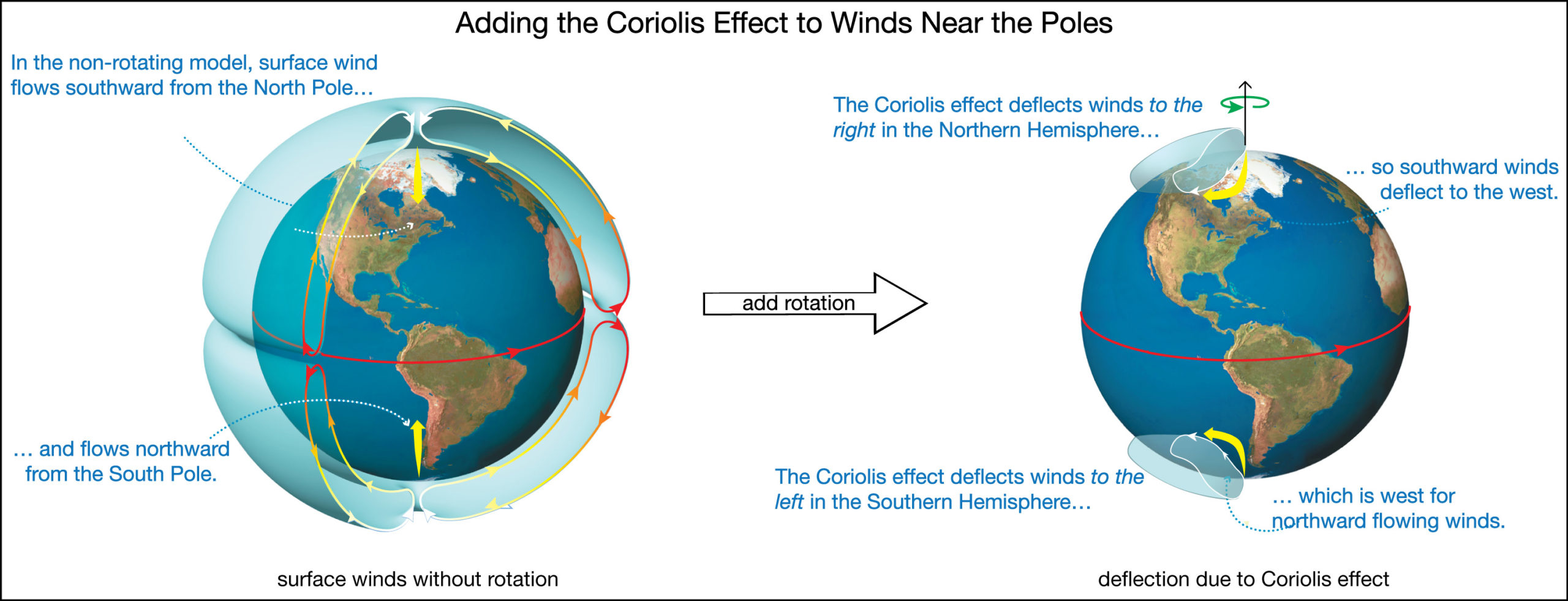
Be sure you notice the following key points in Figure 6.2.2–3:
- Without rotation, surface winds would have flowed continuously all the way from the poles to the equator.
- But Earth does rotate, leading to a Coriolis effect that causes winds headed toward the equator to be deflected toward the west.
- Therefore, the surface winds flowing from the poles are diverted to the west long before they can reach the equator.
Figure 6.2.2–4 shows the result with the added rotation. Each of the large circulation cells of the non-rotating model ends up splitting into three smaller cells in a rotating model. That is, instead of the one large cell of the non-rotating model, each hemisphere has the following:
- an equatorial cell , which extends from the equator to about 30° latitude in each hemisphere.
- a mid-latitude cell , which extends roughly between latitudes 30° to 60° in each hemisphere.
- a polar cell, which extends from about 60° latitude to the pole in each hemisphere.
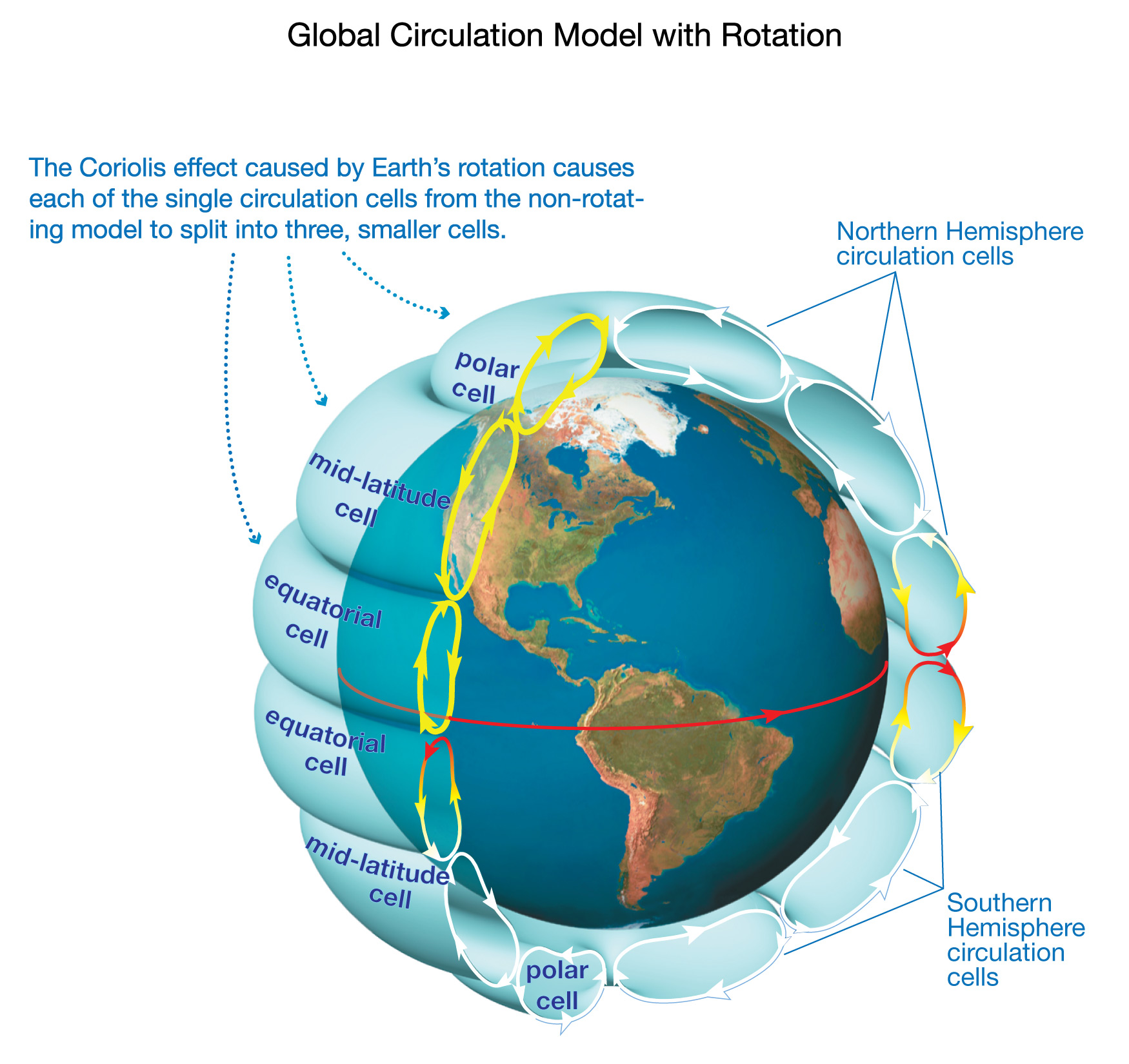
The fact that Earth has a set of three circulation cells in each hemisphere will turn out to explain a lot about winds and climate. Before you continue, try the following quiz to make sure you understand the patterns shown for this three-cell model.
Quiz – Understanding Air Flow in the Three-Cell Model
Answer the following questions based on three-cell model of Figure 6.2.2–4.
Note: As always, after you finish the quiz, be sure you read the feedback that explains the correct answers, to make sure you understand the reasons for these answers.
The purpose of the above quiz is to make sure that students understand how to interpret the arrows on the circulation cells of Figure 6.2.2–4. Note that:
- Questions 1 and 2 make sure they understand the surface flow directions, which we will use immediately below to explain the global wind pattern.
- Questions 3 and 4 consider upward and downward flowing air. Some students may already realize that we can connect these air flows to high and low pressure latitude bands on Earth (as illustrated in Figure 6.2.1–10). However, we will save this discussion until Section 6.3.2, where we will connect it to the locations of different climate zones on Earth.
Explaining Surface Wind Directions
Recall that, as you saw in Figure 6.2.2–1, equatorial and polar winds generally blow from east to west while mid-latitude blow from west to east. The following question set should help you understand how the three-cell circulation model explains this general wind pattern.
Discuss the following questions with a classmate. Then click to open the answers to see if they agree with what you came up with.
1. In the previous question set, you found that the Coriolis effect causes winds flowing toward the equator to be diverted to the west in both hemispheres. In which direction are winds flowing away from the equator diverted? Explain why, using the fact that the Coriolis effect diverts winds to the right in the Northern Hemisphere and to the left in the Southern Hemisphere.
Winds flowing away from the equator are deflected to the east in both hemispheres. You can understand why as follows. In the Northern Hemisphere, winds flowing away from the equator are going north and are deflected to the right, which means to the east. In the Southern Hemisphere, winds flowing away from the equator are going south and are deflected to the left, which also means to the east.
2. Based on your answer to the first question and the directions of surface wind flow in the three cell sets shown in Figure 6.2.2–4 (equatorial, mid-latitude, and polar), in which cells are surface winds deflected to the east?
3. In which of the three cell sets shown in Figure 6.2.2–4 (equatorial, mid-latitude, and polar) are surface winds deflected to the west?
Winds are diverted toward the west in the cells in which air flow along the surface is going toward the equator, which means in both the equatorial and polar cells.
Figure 6.2.2–5 summarizes what you should have found when answering the above questions. Notice that it shows the same alternating wind directions as Figure 6.2.2–1, but now with the added detail of the deflections that shape the patterns.
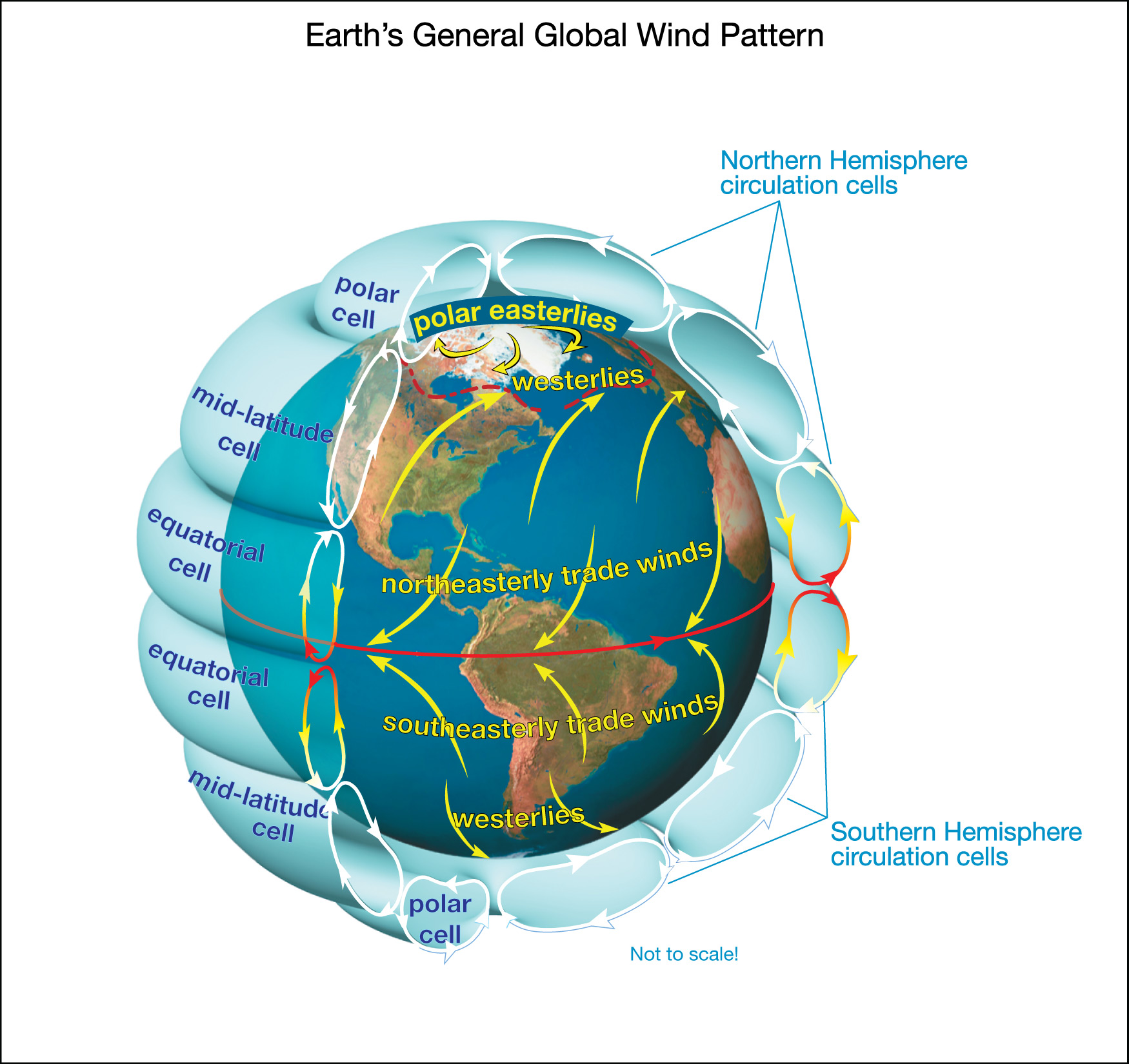
Figure 6.2.2–5 also shows common names given to the sets of winds. These names should make sense based on what you have already learned. Let’s go through them, proceeding from the poles to the equator.
- The winds in the polar cells flow generally from east to west, which is why they are called polar easterly winds.
- Winds in the mid-latitude cells flow from west to east, making them westerly winds.
- Like the polar winds, equatorial winds are easterly in flowing from east to west. However, they are given the name trade winds because they were so important in the past to sailing ships engaged in trade across the oceans. Those on the north side of the equator are more specifically called northeasterly trade winds (because they blow from the northeast) and those south of the equator are the southeasterly trade winds (because they blow from the southeast).
So there you have it. You now know and understand Earth’s general global wind pattern, which is driven by the first two of the four major drivers of Earth’s winds that we discussed in Section 6.2.1: differences in solar energy input and the Coriolis effect that arises from Earth’s rotation. All the rest of the details of Earth’s winds come from other drivers of winds, especially from the other two major factors we discussed: local variations in air pressure and the effects of surface topography.
Discussion
Global Wind Patterns
Discuss the following questions in small groups or as a class.
- If you follow weather for the USA, you’ll know that storms that hit the west coast (Washington, Oregon, California) generally then move inland to hit the Rocky Mountain states, and often then continue toward the east coast. How do Earth’s global winds explain this storm pattern?
- Suppose you want to make a roundtrip sailing trip across the Atlantic Ocean. Should you follow the same route in both directions? Explain why or why not.
- Would your answers to question 2 change if you were sailing across the Pacific Ocean rather than the Atlantic Ocean? Explain why or why not.
- Challenge question: Figure 6.2.2–5 shows the average global wind pattern on Earth, which has the two sets of trade winds meeting at the equator. In fact, the place where these trade winds meet actually varies with the seasons. Explain why.
This discussion should help reinforce the general wind pattern in students’ minds.
- (1) The USA is at mid-latitudes, where the general wind pattern is westerly (blowing from west to east). That is why storms track in this same direction.
- (2) You would not follow the same route in both directions, because you would want to try to sail with the wind. This means you’d want to use the trade winds when going westward and the westerlies when going eastward, which means you’d want to sail at different latitudes in the two different directions.
- (3) You would use the same pattern in the Pacific, because the global wind patterns are global, meaning they are the same over both oceans.
- (4) To answer this question, students should remember that the first driver of the global circulation is solar energy input, and the latitude of greatest input depends on the season: It is north of the equator during Northern Hemisphere summer, and south of the equator during Southern Hemisphere summer. In other words, if you look at the non-rotating model of Figure 6.2.2–2, the convergence at the equator is the year-round average, but the actual convergence location would be to the north during Northern Hemisphere summer and to the south during Southern Hemisphere summer. This same idea extends to the three-cell model, so that trade winds converge north of the equator during Northern Hemisphere summer and south of the equator during Southern Hemisphere summer.
The latitude at which the trade winds converge is called the intertropical convergence zone (ITCZ), and it is characterized by very weak winds (hence the name “doldrums” used by sailors).
The seasonal change in the latitude of the intertropical convergence zone also explains the shift in rainfall patterns in the tropics over the course of each year, with rainy and dry seasons. We’ll discuss the ITCZ further in Section 6.3.2.