Mass-Energy (E = mc2)
In the process of discovering his special theory of relativity, Einstein also discovered that mass itself is a form of potential energy, often called mass-energy. The amount of potential energy contained in mass is described by Einstein’s famous equation
Because the speed of light is very fast (300,000 kilometers per second, which is the same as 300,000,000 meters per second), the number c2 is a very large number. Therefore, Einstein’s equation tells us that a small amount of mass contains a huge amount of stored energy. For example, the energy released by a 1-megaton H-bomb comes from converting only about 0.1 kilogram of mass (about 3 ounces—a quarter of a can of soda) into energy.
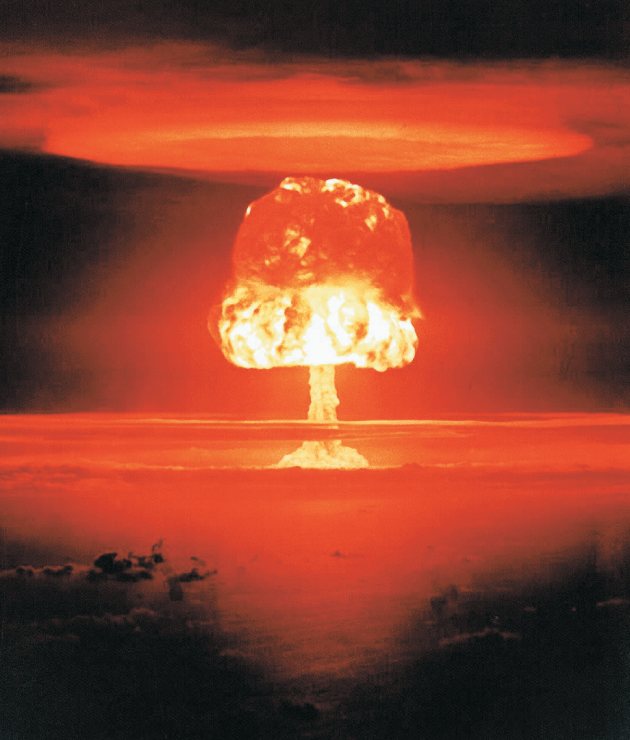
However, even though mass contains an enormous amount of energy, only in relatively rare circumstances is this energy “useful” in the sense of causing movement, heating, or transformation into other forms of energy. The reason is that mass generally stays unchanged. In nature, the only major exceptions occur in:
- Radioactive decay , in which an atomic nucleus undergoes changes that result in the conversion of a small amount of the nucleus’s mass into energy.
Note that one form of radioactive decay is nuclear fission, in which a large atomic nucleus (such as uranium) is split apart into smaller nuclei. Humans have learned to induce fission, and it is the energy source used in nuclear bombs and also in all current nuclear power plants. - Nuclear fusion, which is the power source of stars: The fusion process combines (fuses together) small atomic nuclei to make larger ones, again with a small amount of the original mass being converted to energy in the process. Humans have learned to use fusion in hydrogen bombs (H-bombs), but have not yet developed it as a power source.
As we’ve discussed, radioactive decay is a source of heating for Earth’s interior, but other than that we can generally ignore mass-energy in Earth science.
Three More Notes
For those who really want to “take it to the next level,” here are three more notes you might find interesting about Einstein’s formula E = mc2.
Note 1: You can use the formula to calculate the mass-energy contained in any amount of mass. If you use standard units, then you should put the mass m in kilograms, the speed of light in meters per second (c = 3 108 m/s), and the calculation will then give the energy E in joules.
Example: Suppose that you could somehow convert a 1 kilogram rock completely into energy. How much energy would it release?
Solution: We simply apply the formula with m = 1 kg:
This is an enormous amount of energy; in fact, we’d need to burn about 7.5 billion liters of gasoline to generate the same amount of energy for cars, which is as much gasoline as used by all cars in the United States combined for about a week.
Note 2: As discussed above, radioactive decay, nuclear fission, and nuclear fusion all convert only a small fraction of the original mass into energy. So you might wonder: Are there any processes that can completely convert mass into energy? The answer is yes: There is one process that completely converts mass into energy, and it is called matter–antimatter annihilation. The term antimatter refers to subatomic particles that are sort of like mirror opposites of ordinary matter particles. For example, an antielectron (also called a positron), is just like an ordinary electron except it has a positive electrical charge instead of a negative electrical charge. When any particle of matter meets its corresponding particle of antimatter (such as an electron meeting an antielectron), the particles completely annihilate each other, converting all of the mass into energy.
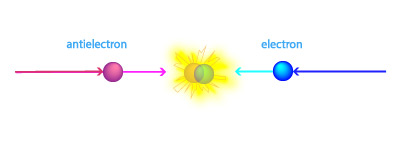
Note 3: Although we more commonly think of E = mc2 as telling us how much energy we can get from mass, the fact that it has an “=” sign tells us that mass and energy can be converted in either direction. In fact, if you can concentrate enough energy in a very small space, the energy can spontaneously turn into subatomic particles of mass. This is why physicists build the gigantic machines known as particle accelerators, such as the Large Hadron Collider in Europe: They accelerate known particles (such as electrons or protons) to extremely high speeds, so that collisions between them release highly concentrated bursts of energy. In the aftermath of these collisions, some of the released energy spontaneously turns into new particles, and sometimes these new particles are of a type that had never before been discovered.
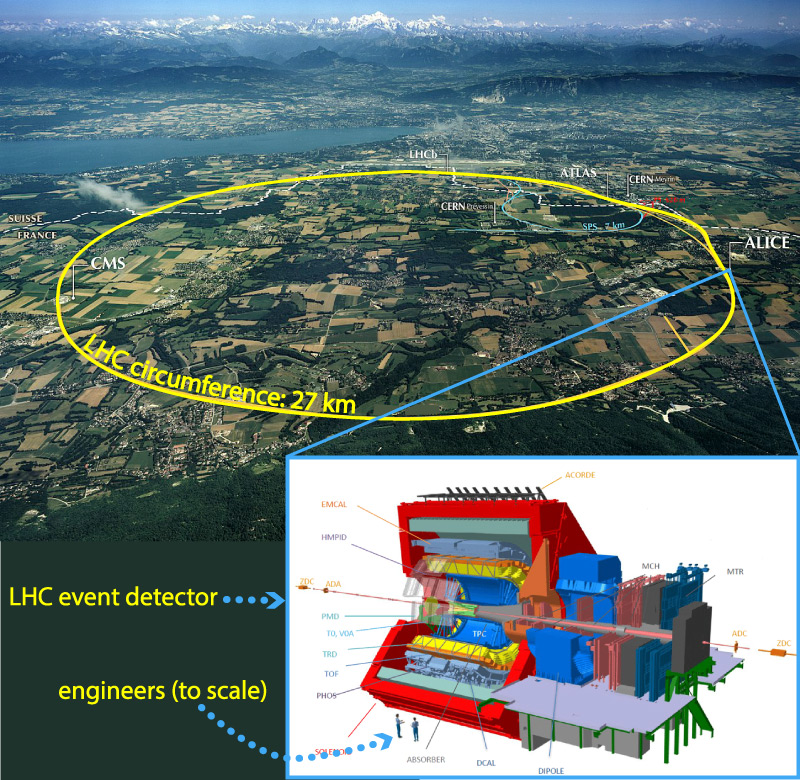