Gravity is a “fact” in the sense that there is no doubt that it is real and does many things. For example, gravity really does hold us to Earth, it really does make objects fall when you drop them, and it really does keep Earth and other planets in orbit around the Sun.
But why does gravity do these things? This is where a theory of gravity comes in, by which we mean a model that can explain the many roles of gravity and predict how gravity will act in other situations. Historically, it took a very long time for an adequate theory of gravity to be developed, and our theory of gravity may still be incomplete even today.
Ancient Ideas about Gravity
In ancient times, the concept of gravity meant nothing more than the tendency of objects to fall to the ground. Because the ancient Greeks recognized that the world is round, they also recognized that gravity acted in a direction toward the center of the Earth. In other words, gravity gave meaning to the notions of “up” and “down” (Figure 3.18).
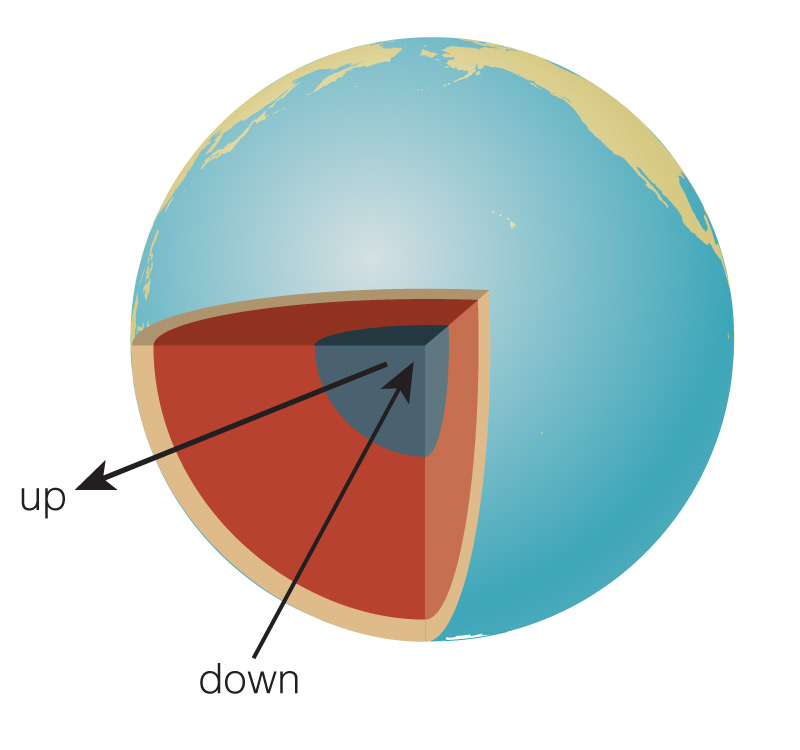
Notice that this implies at least two major differences between the ancient idea of gravity and our modern ideas:
- The ancient idea of gravity applied only to objects on Earth. As far as we know, the idea that gravity might also affect the Moon, planets, or stars never occurred to anyone in the ancient world. They simply assumed that “the heavens” (which is what they called everything in the sky) operated by different rules than Earth.
- People of the ancient world never developed a clear understanding of exactly how gravity affects falling objects. For example, it was commonly assumed that heavier objects must fall faster than lighter-weight objects. This idea wasn’t clearly disproven until Galileo demonstrated that, aside from effects of air resistance, gravity makes all objects fall to the ground at the same rate (Figure 3.19).
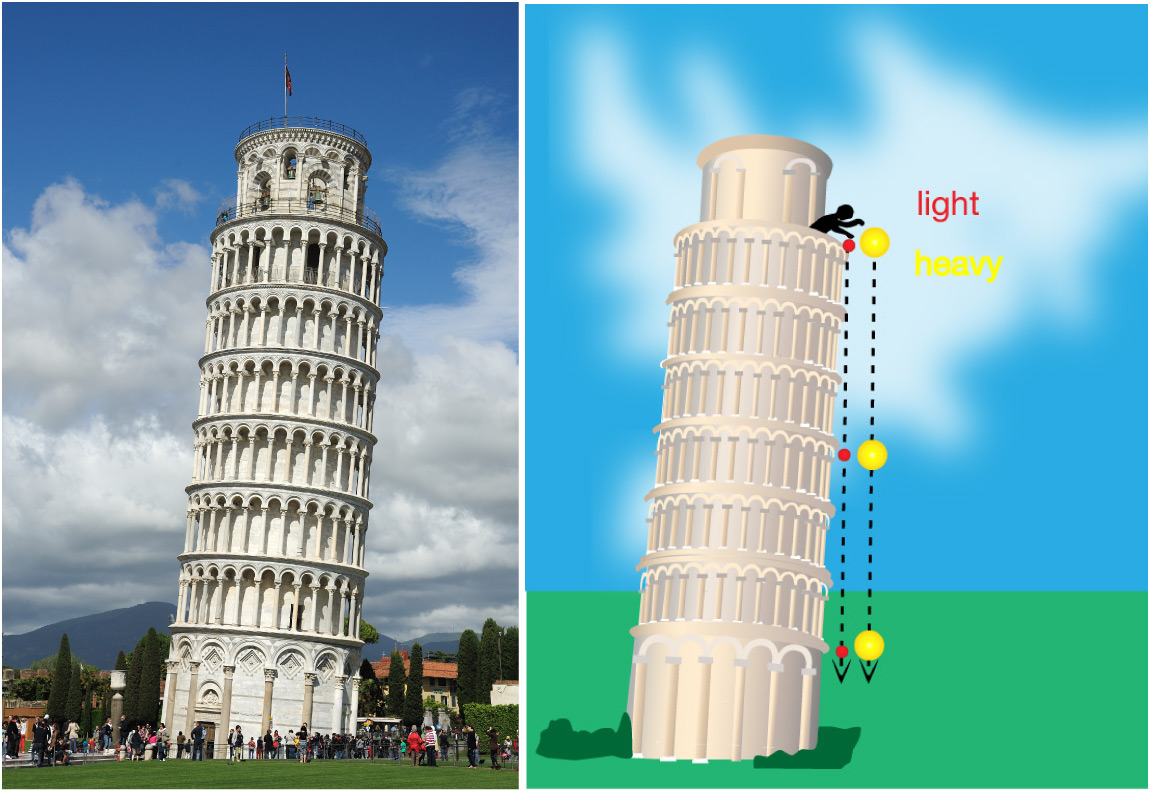
Video
Video. Absolute proof that objects fall at the same rate without air resistance can be found by dropping objects on the Moon, where there is no air. This video shows Apollo 15 astronaut Dave Scott doing this demonstration with a hammer and a feather. Notice that both fall to the ground at precisely the same rate.
Claim-Evidence-Reasoning Activity
Paper and Rock
Find a piece of paper and a small rock. Hold both at the same height, one in each hand, and let them go at the same instant. The rock, of course, hits the ground first. Next, crumple the paper into a small ball and repeat the experiment. Construct a scientific argument, using the Claim-Evidence-Reasoning framework, that uses your results from this experiment to support the following claim:
This simple activity can be done by each student individually, though the Claim-Evidence-Reasoning can be done as a group using the standard rubric. The key point is to recognize that the paper weighs less than the rock regardless of whether it is flat or crumpled. Therefore, since the crumpled paper will fall at the same rate as the rock, we can conclude that the only reason the flat paper fell more slowly must have been the effects of air resistance.
- Be sure that students also recognize how an experiment like this allows us to infer what would happen in the absence of air resistance, even though we cannot make it completely go away in this case.
Connections—Etymology
gravis
Our English word “gravity” has its roots in two Latin words: gravis, which means “heavy,” and gravitis, which means “weight” (also “seriousness”). You’ll notice that these Latin terms seem to reflect the ancient misunderstanding of the role of weight in how fast objects fall. You may also notice how these historical meanings affect other common words and phrases. Think, for example, about what it means when someone says that we face a “grave threat” or refers to “the gravity of the situation.” Can you think of other cases in which the Latin roots of “gravity” appear in other words and phrases?
Coming up with other cases of the same roots may be a bit challenging, but two relatively well-known ones are the following:
-
- aggravate: literally means “to make heavier,” using the root gravis.
- gravitas: this word is commonly used in the sense of a person who “has gravitas” meaning a person who conveys a sense of seriousness, as in the root gravitis.
You can find other though less familiar examples at this web link: http://www.english-for-students.com/Grav.html
Newton’s Theory of Gravity
Galileo’s discovery was an important step in understanding gravity, but it still did not give us a model of gravity, because it didn’t explain why objects of different weights should all fall at the same rate. Moreover, neither Galileo nor anyone else had yet recognized any connection between gravity and planetary motion.
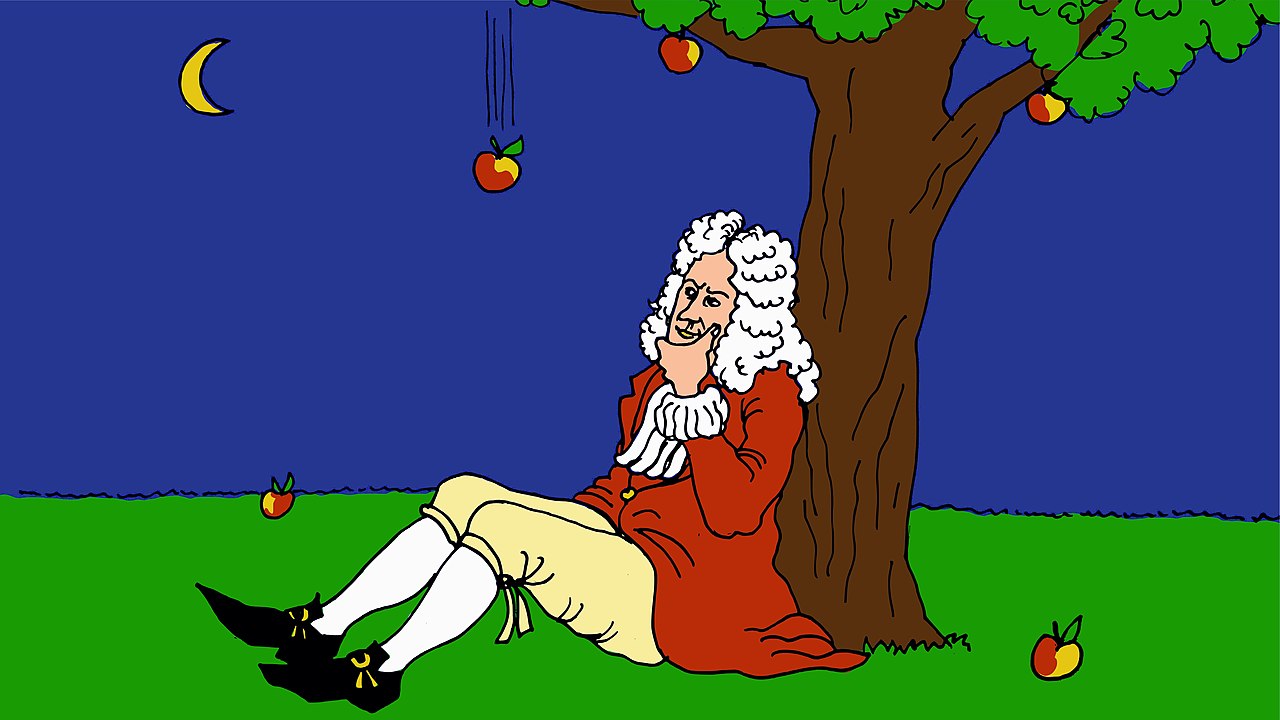
The breakthrough in our understanding of gravity came from Isaac Newton a few decades later. By his own account, Newton experienced a moment of inspiration in 1666 when he saw an apple fall to the ground and suddenly realized that the gravity making the apple fall was the same force that held the Moon in orbit around Earth. (Contrary to a popular myth, Newton did not say that the apple hit him on the head.) But where did this insight come from? To understand it, we have to think back to Kepler’s model of planetary motion, which is embodied in his three laws of planetary motion.
Recall that Kepler had published his first two laws of planetary motion in 1610, and his third law in 1619, and that these laws were clearly successful at predicting planetary positions in the sky. However, for decades afterward, no one could explain why Kepler’s model worked so well, though many scientists recognized that the laws contained two key clues to whatever might explain planetary motion:
- The first clue came from the fact that orbits were ellipses rather than perfect circles. Clearly, whatever caused planetary motion must make it possible for orbits to have these elliptical shapes.
- The second clue came from the fact that planets farther from the Sun orbit at slower speeds (according to the precise mathematical formula of Kepler’s third law). This suggested that the Sun might be the source of whatever force held the planets in their orbits, and that this force weakened with distance, which would explain why more distant planets moved more slowly.
The second clue was particularly important, and many scientists tried to identify the force that might explain planetary motion. There were many guesses; for example, some scientists wondered whether the planets might be held in their orbits by some sort of magnetic force from the Sun.
Claim-Evidence-Reasoning Activity
Gravitational Force
Working in small groups, discuss how Kepler’s laws provided clues about the nature of the force that causes planets to orbit the Sun. Then use evidence and reasoning to support the following claim:
Claim: The fact that planets farther from the Sun orbit at slower speeds implies that the force holding them in orbit weakens with distance from the Sun.
Teacher’s notes: Students may find a variety of ways to support this claim, but some key notes:
- The key evidence to cite is the decline in orbital speed with distance from the Sun.
- The key reasoning is that if the force holding the planets in orbit is emanating from the Sun, then the decline in orbital speeds implies a decline in the force.
- Note that this fact is actually geometrically implicit in the fact that Newton’s universal law of gravitation is an inverse square law. You can think of the force spreading out over a larger and larger area with distance. Because area always scales with the square of distance (in this case, think of the surface area of a sphere, centered on the Sun, and how that surface area changes as the sphere is expanded to different distances from the Sun), the strength of the force would be expected to decline by an inverse square law. (Note: On a fundamental physics level this is exactly the case, since the gravitational force is carried by particles called gravitons that must spread out over an increasing large area with distance.)
From this standpoint, if Newton had simply said “I think it’s gravity,” it would likely have been seen as just another guess. But Newton didn’t stop there; instead, he put mathematics to work and didn’t announce his conclusion that the force was gravity until he had proved it beyond any reasonable doubt.
This work took a while, and also required him to formalize a set of laws describing motion (those that we call Newton’s laws of motion) and to invent a new type of mathematics, called calculus, to work out the details. He finally published his work on motion and gravity in 1687 — more than 20 years after his insight with the falling apple (Figure 3.20).
I was wondering...
What is Calculus?
Coming Soon.
The Universal Law of Gravitation
Key Concepts: The Universal Law of Gravitation
In statement form:
- Every mass attracts every other mass through the force called gravity.
- The strength of the gravitational force attracting any two objects is directly proportional to the product of their masses. For example, doubling the mass of one object doubles the force of gravity between the two objects.
- The strength of gravity between two objects decreases with the square of the distance between their centers. We therefore say that the gravitational force follows an inverse square law with distance. For example, doubling the distance between two objects weakens the force of gravity by a factor of 22 = 4.
In equation form:
where Fg is the force of gravitational attraction, M1 and M2 are the masses of the two objects, and d is the distance between their centers (Figure 3.21). The symbol G represents a special number called the gravitational constant. (Its numerical value has been measured to be
G =6.67 x 10-11 m3/(kg x s2)
Figure 3.21 provides a summary of the universal law of gravitation in both its statement and mathematical forms.
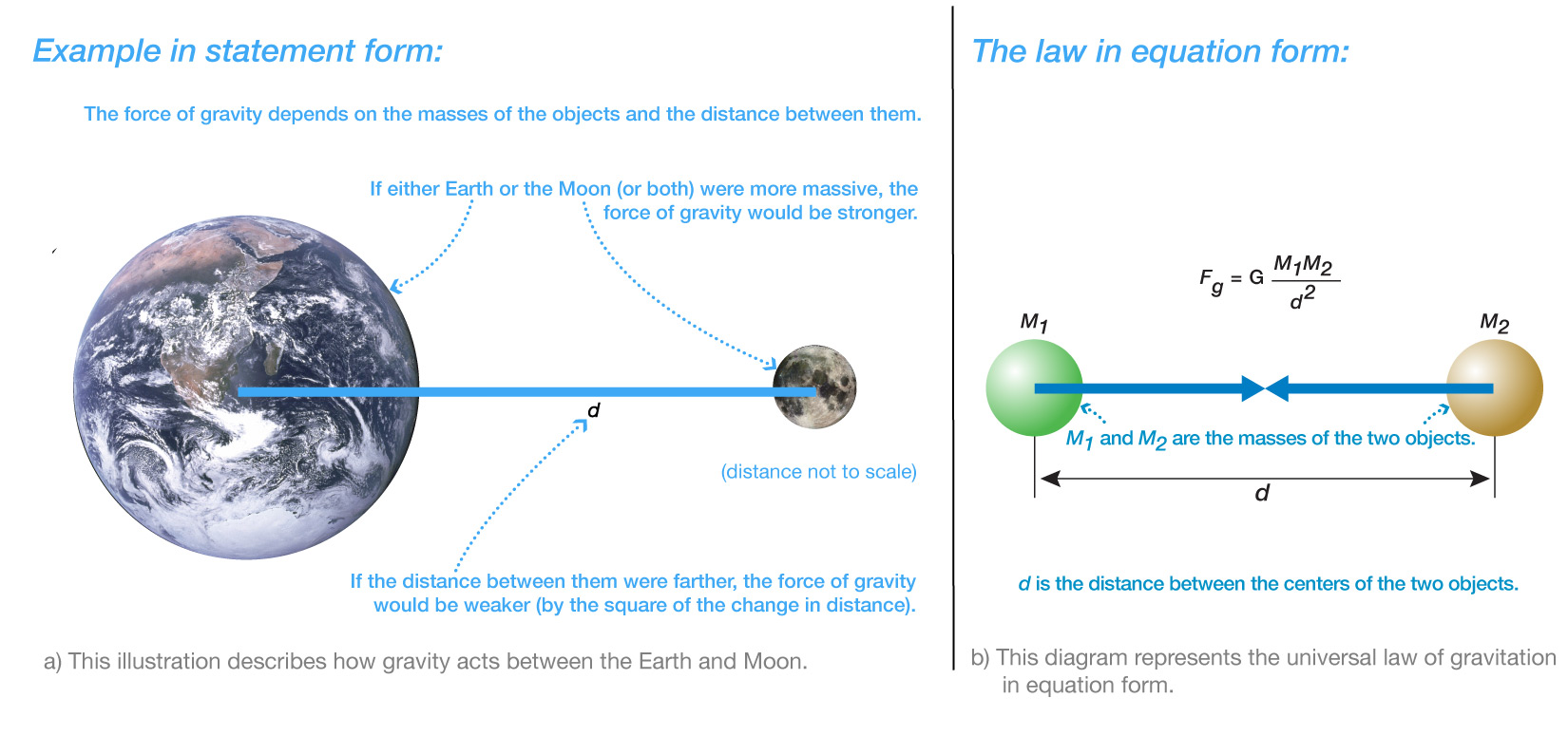
We’ve put the universal law of gravitation in a key concept box since it is so important. Note that we have included both statement and equation forms. You can consider the introduction of the equation to be optional, as many of your students will not yet have learned the algebraic skills to fully understand it. However, for those who have those skills, the equation is actually easier to follow than the verbal statements. And you may find that it’s easy to explain the terms of the equation anyway, with the additional benefit that it will help students who are unfamiliar with the algebraic notation to realize that it’s not really that complicated, which may help them in math later.
Quick Quiz
Note: As always, after you finish the quiz, be sure you read the feedback that explains the correct answers, to make sure you understand the reasons for these answers.
Before we continue, it’s worth noting three important ideas that you should keep in mind about Newton’s universal law of gravitation:
- This law represents a model of gravity, because it can be used to calculate the strength of gravity in any situation and thereby to predict how objects will move as a result of gravity. This model has proven to be extremely successful, and it is this success that leads us to consider it a theory of gravity.
- Newton’s successful development of a theory of gravity represented the culmination of the Copernican revolution. Once Newton demonstrated that gravity explained why planets orbit the Sun as they do, there could be no further doubt that Earth really is a planet orbiting the Sun.
- His discovery that the same law of gravity applies to falling apples and to orbits in space provided the first evidence of the idea that the same laws of nature operate everywhere. In other words, Newton was the first to show that Earth and “the heavens” were not distinct realms, but all part of the same universe, governed by the same laws of nature.
Connections—Etymology and History
Newton Discovered the Universe!
Have you ever thought about what the word “universe” actually means? It’s easy to break down: the root uni means “one,” and verse means “story.” In other words, the word “universe” means “one story.” In this sense, Newton’s discovery of the law of gravity also represented the discovery of the universe. Before that, it was generally believed that Earth and “the heavens” were two completely distinct realms. By proving that the same law of gravity applied both on Earth and in space, he showed that we no longer needed to think about two distinct realms, but could instead understand both as being part of the same “one story” – the universe.
Group Activity
Copernican Revolution Timeline
We’ve covered a lot of dates in this chapter relating to the Copernican revolution. Working in small groups, go back through the chapter to find all the dates and the related significant events, starting back in 500 BC when Pythagoras was known to be teaching that Earth is round. Put all the dates on a timeline, with brief labels for the significant events. Be sure to use a consistent scale for your timeline; you can choose any scale you wish, but note that the length of the timeline will depend on the scale you choose. For example, if you use a scale of 1 cm = 50 years, then making a timeline for 2,500 years will require a sheet of paper (or several sheets taped together) that is 50 centimeters long.
Teacher Notes: This optional activity will help students understand the historical context of the Copernican revolution. It will work best with a long sheet of paper (or many individual sheets taped together). Encourage students to use a consistent scale, such as 1 inch = 50 years, in which case the 2,200 year period from Pythagoras to Newton will require a sheet of paper that is 44 inches long (which can be made by taping together four 8.5×11 inch sheets). Note that there will be significant stretches with nothing marked, but a lot of action toward the end. Students should work in small groups, so they can divide up the task of identifying all the key events covered in this chapter. Key events that should be listed include:
- 500 B.C.: Pythagoras teaches that Earth is round.
- 240 B.C.: Eratosthenes calculates the circumference of the Earth.
- 150 A.D.: Ptolemy publishes Earth-centered model.
- 1543 : Copernicus publishes De Revolutionibus Orbiium Ceolestium.
- 1546-1601: Tycho Brahe makes most detailed observations to date.
- 1600: Brahe hires Kepler.
- 1609: Kepler publishes 1st and 2nd laws.
- 1610: Galileo observes moons of Jupiter, phases of Venus and stars in Milky Way.
- 1619: Kepler publishes 3rd law.
- 1633: trial of Galileo, sun-centered model almost universally accepted.
- 1687: Newton publishes universal law of gravitation.
Do the Math
How Newton Explained Galileo’s Discovery
coming soon
Gravity Beyond Newton
Newton’s theory of gravity works so well that it might be tempting to think that it represents everything we need to know about gravity. But it doesn’t. In the centuries after Newton, scientists discovered that the predictions of Newton’s theory are not quite perfect, particularly in cases where gravity is very strong. As a result, scientists kept working to understand gravity better.
Today our best theory of gravity is a theory first developed by Albert Einstein (1879–1955), called the general theory of relativity. In other words, despite its odd-sounding name, “general relativity” (as it is usually called) is actually a theory of gravity. The reason that we consider general relativity to be a better theory is because when we measure with high enough precision, we find that Einstein’s general theory of relativity makes predictions that differ very slightly from Newton’s theory — and the observations agree better with Einstein’s theory. Moreover, in some cases, general relativity makes predictions that go far beyond the bounds of Newton’s theory. For example, general relativity predicts the existence of both black holes and of something called “gravitational waves,” both of which have now been observed to exist just as predicted (Figures 3.22 and 3.23).
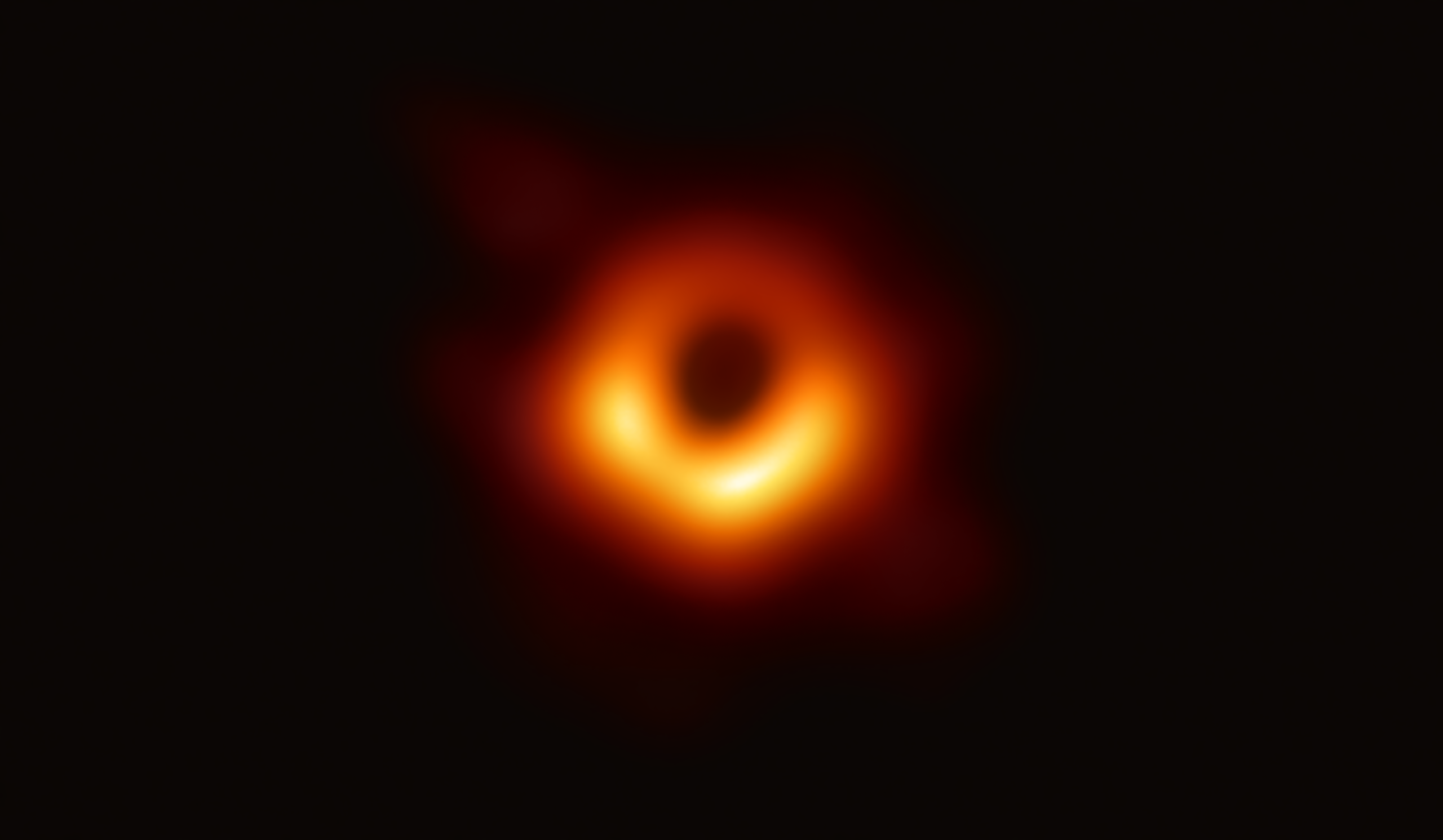
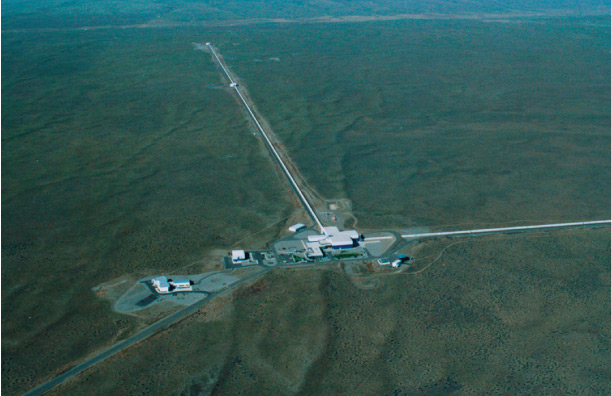
Does this mean that Newton’s theory of gravity was “wrong”? Remember that Newton’s theory successfully explains nearly all observations of gravity in the universe, and it works so well that we can use it to plot the courses of spacecraft to the planets. Moreover, in all cases in which Newton’s theory works well, Einstein’s theory gives essentially the same answers. The differences in the predictions between the two theories are noticeable only with extremely precise measurements or in cases where gravity is unusually strong.
We therefore do not say that Newton’s theory was wrong, but rather that it is only an approximation to a more exact theory of gravity—Einstein’s general theory of relativity. Under most circumstances, the approximation is so good that we can barely tell the difference between the two theories of gravity, but in cases of strong gravity, Einstein’s theory works and Newton’s fails.
Video
Do you want to learn more about Einstein’s theory of gravity? This hour-long video presentation by the textbook author introduces both Einstein’s special and general theories of relativity.
Summary: Gravity as Fact and Theory
We can now summarize the distinction between talking about gravity as a fact and as a theory:
- The fact of gravity is obvious in the observations we make of falling objects on Earth and of orbiting objects in space. There is no doubt that gravity really exists.
- A theory of gravity is a specific model of gravity that we use to make predictions that we then put to the test through observations or experiments.
Newton provided our first theory of gravity, and it still works extremely well in almost all cases. However, it is not quite perfect, and our best theory of gravity today is Einstein’s general theory of relativity. In the future, our theory of gravity may be further improved, but gravity remains a fact regardless of how we revise the theory.
Discussion
Facts and Theories
At the beginning of this section, you wrote down answers to three questions about what we mean in saying that gravity is both a fact and a theory. Look back at your answers, then do the following:
- Discuss whether you still like the answers you wrote earlier, or think they should be modified. If you think they should be modified, make the modifications.
- Gravity is not unique in being something that we talk about in terms of both fact and theory. Try to think of at least two other similar cases, and describe the distinction between fact and theory in each case.
The first part of the discussion below simply revisits their earlier discussion. For (2), a few of the cases that students might think of:
- Atoms: The existence of atoms is an observed fact, but we use atomic theory to explain why atoms behave as they do.
- Evolution: The fossil record reveals the fact that evolution has occurred, but Darwin’s theory of evolution by natural selection explains why evolution occurs.
- …[other examples]